mirror of
git://git.tartarus.org/simon/puzzles.git
synced 2025-04-21 16:05:44 -07:00
Files
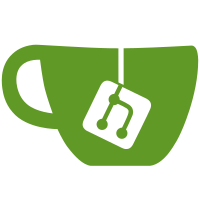
is mostly done with ifdefs in windows.c; so mkfiles.pl generates a new makefile (Makefile.wce) and Recipe enables it, but it's hardly any different from Makefile.vc apart from a few definitions at the top of the files. Currently the PocketPC build is not enabled in the build script, but with any luck I'll be able to do so reasonably soon. [originally from svn r7337]
2182 lines
62 KiB
C
2182 lines
62 KiB
C
/*
|
|
* tents.c: Puzzle involving placing tents next to trees subject to
|
|
* some confusing conditions.
|
|
*
|
|
* TODO:
|
|
*
|
|
* - error highlighting?
|
|
* * highlighting adjacent tents is easy
|
|
* * highlighting violated numeric clues is almost as easy
|
|
* (might want to pay attention to NONTENTs here)
|
|
* * but how in hell do we highlight a failure of maxflow
|
|
* during completion checking?
|
|
* + well, the _obvious_ approach is to use maxflow's own
|
|
* error report: it will provide, via the `cut' parameter,
|
|
* a set of trees which have too few tents between them.
|
|
* It's unclear that this will be particularly obvious to
|
|
* a user, however. Is there any other way?
|
|
*
|
|
* - it might be nice to make setter-provided tent/nontent clues
|
|
* inviolable?
|
|
* * on the other hand, this would introduce considerable extra
|
|
* complexity and size into the game state; also inviolable
|
|
* clues would have to be marked as such somehow, in an
|
|
* intrusive and annoying manner. Since they're never
|
|
* generated by _my_ generator, I'm currently more inclined
|
|
* not to bother.
|
|
*
|
|
* - more difficult levels at the top end?
|
|
* * for example, sometimes we can deduce that two BLANKs in
|
|
* the same row are each adjacent to the same unattached tree
|
|
* and to nothing else, implying that they can't both be
|
|
* tents; this enables us to rule out some extra combinations
|
|
* in the row-based deduction loop, and hence deduce more
|
|
* from the number in that row than we could otherwise do.
|
|
* * that by itself doesn't seem worth implementing a new
|
|
* difficulty level for, but if I can find a few more things
|
|
* like that then it might become worthwhile.
|
|
* * I wonder if there's a sensible heuristic for where to
|
|
* guess which would make a recursive solver viable?
|
|
*/
|
|
|
|
#include <stdio.h>
|
|
#include <stdlib.h>
|
|
#include <string.h>
|
|
#include <assert.h>
|
|
#include <ctype.h>
|
|
#include <math.h>
|
|
|
|
#include "puzzles.h"
|
|
#include "maxflow.h"
|
|
|
|
/*
|
|
* Design discussion
|
|
* -----------------
|
|
*
|
|
* The rules of this puzzle as available on the WWW are poorly
|
|
* specified. The bits about tents having to be orthogonally
|
|
* adjacent to trees, tents not being even diagonally adjacent to
|
|
* one another, and the number of tents in each row and column
|
|
* being given are simple enough; the difficult bit is the
|
|
* tent-to-tree matching.
|
|
*
|
|
* Some sources use simplistic wordings such as `each tree is
|
|
* exactly connected to only one tent', which is extremely unclear:
|
|
* it's easy to read erroneously as `each tree is _orthogonally
|
|
* adjacent_ to exactly one tent', which is definitely incorrect.
|
|
* Even the most coherent sources I've found don't do a much better
|
|
* job of stating the rule.
|
|
*
|
|
* A more precise statement of the rule is that it must be possible
|
|
* to find a bijection f between tents and trees such that each
|
|
* tree T is orthogonally adjacent to the tent f(T), but that a
|
|
* tent is permitted to be adjacent to other trees in addition to
|
|
* its own. This slightly non-obvious criterion is what gives this
|
|
* puzzle most of its subtlety.
|
|
*
|
|
* However, there's a particularly subtle ambiguity left over. Is
|
|
* the bijection between tents and trees required to be _unique_?
|
|
* In other words, is that bijection conceptually something the
|
|
* player should be able to exhibit as part of the solution (even
|
|
* if they aren't actually required to do so)? Or is it sufficient
|
|
* to have a unique _placement_ of the tents which gives rise to at
|
|
* least one suitable bijection?
|
|
*
|
|
* The puzzle shown to the right of this .T. 2 *T* 2
|
|
* paragraph illustrates the problem. There T.T 0 -> T-T 0
|
|
* are two distinct bijections available. .T. 2 *T* 2
|
|
* The answer to the above question will
|
|
* determine whether it's a valid puzzle. 202 202
|
|
*
|
|
* This is an important question, because it affects both the
|
|
* player and the generator. Eventually I found all the instances
|
|
* of this puzzle I could Google up, solved them all by hand, and
|
|
* verified that in all cases the tree/tent matching was uniquely
|
|
* determined given the tree and tent positions. Therefore, the
|
|
* puzzle as implemented in this source file takes the following
|
|
* policy:
|
|
*
|
|
* - When checking a user-supplied solution for correctness, only
|
|
* verify that there exists _at least_ one matching.
|
|
* - When generating a puzzle, enforce that there must be
|
|
* _exactly_ one.
|
|
*
|
|
* Algorithmic implications
|
|
* ------------------------
|
|
*
|
|
* Another way of phrasing the tree/tent matching criterion is to
|
|
* say that the bipartite adjacency graph between trees and tents
|
|
* has a perfect matching. That is, if you construct a graph which
|
|
* has a vertex per tree and a vertex per tent, and an edge between
|
|
* any tree and tent which are orthogonally adjacent, it is
|
|
* possible to find a set of N edges of that graph (where N is the
|
|
* number of trees and also the number of tents) which between them
|
|
* connect every tree to every tent.
|
|
*
|
|
* The most efficient known algorithms for finding such a matching
|
|
* given a graph, as far as I'm aware, are the Munkres assignment
|
|
* algorithm (also known as the Hungarian algorithm) and the
|
|
* Ford-Fulkerson algorithm (for finding optimal flows in
|
|
* networks). Each of these takes O(N^3) running time; so we're
|
|
* talking O(N^3) time to verify any candidate solution to this
|
|
* puzzle. That's just about OK if you're doing it once per mouse
|
|
* click (and in fact not even that, since the sensible thing to do
|
|
* is check all the _other_ puzzle criteria and only wade into this
|
|
* quagmire if none are violated); but if the solver had to keep
|
|
* doing N^3 work internally, then it would probably end up with
|
|
* more like N^5 or N^6 running time, and grid generation would
|
|
* become very clunky.
|
|
*
|
|
* Fortunately, I've been able to prove a very useful property of
|
|
* _unique_ perfect matchings, by adapting the proof of Hall's
|
|
* Marriage Theorem. For those unaware of Hall's Theorem, I'll
|
|
* recap it and its proof: it states that a bipartite graph
|
|
* contains a perfect matching iff every set of vertices on the
|
|
* left side of the graph have a neighbourhood _at least_ as big on
|
|
* the right.
|
|
*
|
|
* This condition is obviously satisfied if a perfect matching does
|
|
* exist; each left-side node has a distinct right-side node which
|
|
* is the one assigned to it by the matching, and thus any set of n
|
|
* left vertices must have a combined neighbourhood containing at
|
|
* least the n corresponding right vertices, and possibly others
|
|
* too. Alternatively, imagine if you had (say) three left-side
|
|
* nodes all of which were connected to only two right-side nodes
|
|
* between them: any perfect matching would have to assign one of
|
|
* those two right nodes to each of the three left nodes, and still
|
|
* give the three left nodes a different right node each. This is
|
|
* of course impossible.
|
|
*
|
|
* To prove the converse (that if every subset of left vertices
|
|
* satisfies the Hall condition then a perfect matching exists),
|
|
* consider trying to find a proper subset of the left vertices
|
|
* which _exactly_ satisfies the Hall condition: that is, its right
|
|
* neighbourhood is precisely the same size as it. If we can find
|
|
* such a subset, then we can split the bipartite graph into two
|
|
* smaller ones: one consisting of the left subset and its right
|
|
* neighbourhood, the other consisting of everything else. Edges
|
|
* from the left side of the former graph to the right side of the
|
|
* latter do not exist, by construction; edges from the right side
|
|
* of the former to the left of the latter cannot be part of any
|
|
* perfect matching because otherwise the left subset would not be
|
|
* left with enough distinct right vertices to connect to (this is
|
|
* exactly the same deduction used in Solo's set analysis). You can
|
|
* then prove (left as an exercise) that both these smaller graphs
|
|
* still satisfy the Hall condition, and therefore the proof will
|
|
* follow by induction.
|
|
*
|
|
* There's one other possibility, which is the case where _no_
|
|
* proper subset of the left vertices has a right neighbourhood of
|
|
* exactly the same size. That is, every left subset has a strictly
|
|
* _larger_ right neighbourhood. In this situation, we can simply
|
|
* remove an _arbitrary_ edge from the graph. This cannot reduce
|
|
* the size of any left subset's right neighbourhood by more than
|
|
* one, so if all neighbourhoods were strictly bigger than they
|
|
* needed to be initially, they must now still be _at least as big_
|
|
* as they need to be. So we can keep throwing out arbitrary edges
|
|
* until we find a set which exactly satisfies the Hall condition,
|
|
* and then proceed as above. []
|
|
*
|
|
* That's Hall's theorem. I now build on this by examining the
|
|
* circumstances in which a bipartite graph can have a _unique_
|
|
* perfect matching. It is clear that in the second case, where no
|
|
* left subset exactly satisfies the Hall condition and so we can
|
|
* remove an arbitrary edge, there cannot be a unique perfect
|
|
* matching: given one perfect matching, we choose our arbitrary
|
|
* removed edge to be one of those contained in it, and then we can
|
|
* still find a perfect matching in the remaining graph, which will
|
|
* be a distinct perfect matching in the original.
|
|
*
|
|
* So it is a necessary condition for a unique perfect matching
|
|
* that there must be at least one proper left subset which
|
|
* _exactly_ satisfies the Hall condition. But now consider the
|
|
* smaller graph constructed by taking that left subset and its
|
|
* neighbourhood: if the graph as a whole had a unique perfect
|
|
* matching, then so must this smaller one, which means we can find
|
|
* a proper left subset _again_, and so on. Repeating this process
|
|
* must eventually reduce us to a graph with only one left-side
|
|
* vertex (so there are no proper subsets at all); this vertex must
|
|
* be connected to only one right-side vertex, and hence must be so
|
|
* in the original graph as well (by construction). So we can
|
|
* discard this vertex pair from the graph, and any other edges
|
|
* that involved it (which will by construction be from other left
|
|
* vertices only), and the resulting smaller graph still has a
|
|
* unique perfect matching which means we can do the same thing
|
|
* again.
|
|
*
|
|
* In other words, given any bipartite graph with a unique perfect
|
|
* matching, we can find that matching by the following extremely
|
|
* simple algorithm:
|
|
*
|
|
* - Find a left-side vertex which is only connected to one
|
|
* right-side vertex.
|
|
* - Assign those vertices to one another, and therefore discard
|
|
* any other edges connecting to that right vertex.
|
|
* - Repeat until all vertices have been matched.
|
|
*
|
|
* This algorithm can be run in O(V+E) time (where V is the number
|
|
* of vertices and E is the number of edges in the graph), and the
|
|
* only way it can fail is if there is not a unique perfect
|
|
* matching (either because there is no matching at all, or because
|
|
* it isn't unique; but it can't distinguish those cases).
|
|
*
|
|
* Thus, the internal solver in this source file can be confident
|
|
* that if the tree/tent matching is uniquely determined by the
|
|
* tree and tent positions, it can find it using only this kind of
|
|
* obvious and simple operation: assign a tree to a tent if it
|
|
* cannot possibly belong to any other tent, and vice versa. If the
|
|
* solver were _only_ trying to determine the matching, even that
|
|
* `vice versa' wouldn't be required; but it can come in handy when
|
|
* not all the tents have been placed yet. I can therefore be
|
|
* reasonably confident that as long as my solver doesn't need to
|
|
* cope with grids that have a non-unique matching, it will also
|
|
* not need to do anything complicated like set analysis between
|
|
* trees and tents.
|
|
*/
|
|
|
|
/*
|
|
* In standalone solver mode, `verbose' is a variable which can be
|
|
* set by command-line option; in debugging mode it's simply always
|
|
* true.
|
|
*/
|
|
#if defined STANDALONE_SOLVER
|
|
#define SOLVER_DIAGNOSTICS
|
|
int verbose = FALSE;
|
|
#elif defined SOLVER_DIAGNOSTICS
|
|
#define verbose TRUE
|
|
#endif
|
|
|
|
/*
|
|
* Difficulty levels. I do some macro ickery here to ensure that my
|
|
* enum and the various forms of my name list always match up.
|
|
*/
|
|
#define DIFFLIST(A) \
|
|
A(EASY,Easy,e) \
|
|
A(TRICKY,Tricky,t)
|
|
#define ENUM(upper,title,lower) DIFF_ ## upper,
|
|
#define TITLE(upper,title,lower) #title,
|
|
#define ENCODE(upper,title,lower) #lower
|
|
#define CONFIG(upper,title,lower) ":" #title
|
|
enum { DIFFLIST(ENUM) DIFFCOUNT };
|
|
static char const *const tents_diffnames[] = { DIFFLIST(TITLE) };
|
|
static char const tents_diffchars[] = DIFFLIST(ENCODE);
|
|
#define DIFFCONFIG DIFFLIST(CONFIG)
|
|
|
|
enum {
|
|
COL_BACKGROUND,
|
|
COL_GRID,
|
|
COL_GRASS,
|
|
COL_TREETRUNK,
|
|
COL_TREELEAF,
|
|
COL_TENT,
|
|
NCOLOURS
|
|
};
|
|
|
|
enum { BLANK, TREE, TENT, NONTENT, MAGIC };
|
|
|
|
struct game_params {
|
|
int w, h;
|
|
int diff;
|
|
};
|
|
|
|
struct numbers {
|
|
int refcount;
|
|
int *numbers;
|
|
};
|
|
|
|
struct game_state {
|
|
game_params p;
|
|
char *grid;
|
|
struct numbers *numbers;
|
|
int completed, used_solve;
|
|
};
|
|
|
|
static game_params *default_params(void)
|
|
{
|
|
game_params *ret = snew(game_params);
|
|
|
|
ret->w = ret->h = 8;
|
|
ret->diff = DIFF_EASY;
|
|
|
|
return ret;
|
|
}
|
|
|
|
static const struct game_params tents_presets[] = {
|
|
{8, 8, DIFF_EASY},
|
|
{8, 8, DIFF_TRICKY},
|
|
{10, 10, DIFF_EASY},
|
|
{10, 10, DIFF_TRICKY},
|
|
{15, 15, DIFF_EASY},
|
|
{15, 15, DIFF_TRICKY},
|
|
};
|
|
|
|
static int game_fetch_preset(int i, char **name, game_params **params)
|
|
{
|
|
game_params *ret;
|
|
char str[80];
|
|
|
|
if (i < 0 || i >= lenof(tents_presets))
|
|
return FALSE;
|
|
|
|
ret = snew(game_params);
|
|
*ret = tents_presets[i];
|
|
|
|
sprintf(str, "%dx%d %s", ret->w, ret->h, tents_diffnames[ret->diff]);
|
|
|
|
*name = dupstr(str);
|
|
*params = ret;
|
|
return TRUE;
|
|
}
|
|
|
|
static void free_params(game_params *params)
|
|
{
|
|
sfree(params);
|
|
}
|
|
|
|
static game_params *dup_params(game_params *params)
|
|
{
|
|
game_params *ret = snew(game_params);
|
|
*ret = *params; /* structure copy */
|
|
return ret;
|
|
}
|
|
|
|
static void decode_params(game_params *params, char const *string)
|
|
{
|
|
params->w = params->h = atoi(string);
|
|
while (*string && isdigit((unsigned char)*string)) string++;
|
|
if (*string == 'x') {
|
|
string++;
|
|
params->h = atoi(string);
|
|
while (*string && isdigit((unsigned char)*string)) string++;
|
|
}
|
|
if (*string == 'd') {
|
|
int i;
|
|
string++;
|
|
for (i = 0; i < DIFFCOUNT; i++)
|
|
if (*string == tents_diffchars[i])
|
|
params->diff = i;
|
|
if (*string) string++;
|
|
}
|
|
}
|
|
|
|
static char *encode_params(game_params *params, int full)
|
|
{
|
|
char buf[120];
|
|
|
|
sprintf(buf, "%dx%d", params->w, params->h);
|
|
if (full)
|
|
sprintf(buf + strlen(buf), "d%c",
|
|
tents_diffchars[params->diff]);
|
|
return dupstr(buf);
|
|
}
|
|
|
|
static config_item *game_configure(game_params *params)
|
|
{
|
|
config_item *ret;
|
|
char buf[80];
|
|
|
|
ret = snewn(4, config_item);
|
|
|
|
ret[0].name = "Width";
|
|
ret[0].type = C_STRING;
|
|
sprintf(buf, "%d", params->w);
|
|
ret[0].sval = dupstr(buf);
|
|
ret[0].ival = 0;
|
|
|
|
ret[1].name = "Height";
|
|
ret[1].type = C_STRING;
|
|
sprintf(buf, "%d", params->h);
|
|
ret[1].sval = dupstr(buf);
|
|
ret[1].ival = 0;
|
|
|
|
ret[2].name = "Difficulty";
|
|
ret[2].type = C_CHOICES;
|
|
ret[2].sval = DIFFCONFIG;
|
|
ret[2].ival = params->diff;
|
|
|
|
ret[3].name = NULL;
|
|
ret[3].type = C_END;
|
|
ret[3].sval = NULL;
|
|
ret[3].ival = 0;
|
|
|
|
return ret;
|
|
}
|
|
|
|
static game_params *custom_params(config_item *cfg)
|
|
{
|
|
game_params *ret = snew(game_params);
|
|
|
|
ret->w = atoi(cfg[0].sval);
|
|
ret->h = atoi(cfg[1].sval);
|
|
ret->diff = cfg[2].ival;
|
|
|
|
return ret;
|
|
}
|
|
|
|
static char *validate_params(game_params *params, int full)
|
|
{
|
|
/*
|
|
* Generating anything under 4x4 runs into trouble of one kind
|
|
* or another.
|
|
*/
|
|
if (params->w < 4 || params->h < 4)
|
|
return "Width and height must both be at least four";
|
|
return NULL;
|
|
}
|
|
|
|
/*
|
|
* Scratch space for solver.
|
|
*/
|
|
enum { N, U, L, R, D, MAXDIR }; /* link directions */
|
|
#define dx(d) ( ((d)==R) - ((d)==L) )
|
|
#define dy(d) ( ((d)==D) - ((d)==U) )
|
|
#define F(d) ( U + D - (d) )
|
|
struct solver_scratch {
|
|
char *links; /* mapping between trees and tents */
|
|
int *locs;
|
|
char *place, *mrows, *trows;
|
|
};
|
|
|
|
static struct solver_scratch *new_scratch(int w, int h)
|
|
{
|
|
struct solver_scratch *ret = snew(struct solver_scratch);
|
|
|
|
ret->links = snewn(w*h, char);
|
|
ret->locs = snewn(max(w, h), int);
|
|
ret->place = snewn(max(w, h), char);
|
|
ret->mrows = snewn(3 * max(w, h), char);
|
|
ret->trows = snewn(3 * max(w, h), char);
|
|
|
|
return ret;
|
|
}
|
|
|
|
static void free_scratch(struct solver_scratch *sc)
|
|
{
|
|
sfree(sc->trows);
|
|
sfree(sc->mrows);
|
|
sfree(sc->place);
|
|
sfree(sc->locs);
|
|
sfree(sc->links);
|
|
sfree(sc);
|
|
}
|
|
|
|
/*
|
|
* Solver. Returns 0 for impossibility, 1 for success, 2 for
|
|
* ambiguity or failure to converge.
|
|
*/
|
|
static int tents_solve(int w, int h, const char *grid, int *numbers,
|
|
char *soln, struct solver_scratch *sc, int diff)
|
|
{
|
|
int x, y, d, i, j;
|
|
char *mrow, *mrow1, *mrow2, *trow, *trow1, *trow2;
|
|
|
|
/*
|
|
* Set up solver data.
|
|
*/
|
|
memset(sc->links, N, w*h);
|
|
|
|
/*
|
|
* Set up solution array.
|
|
*/
|
|
memcpy(soln, grid, w*h);
|
|
|
|
/*
|
|
* Main solver loop.
|
|
*/
|
|
while (1) {
|
|
int done_something = FALSE;
|
|
|
|
/*
|
|
* Any tent which has only one unattached tree adjacent to
|
|
* it can be tied to that tree.
|
|
*/
|
|
for (y = 0; y < h; y++)
|
|
for (x = 0; x < w; x++)
|
|
if (soln[y*w+x] == TENT && !sc->links[y*w+x]) {
|
|
int linkd = 0;
|
|
|
|
for (d = 1; d < MAXDIR; d++) {
|
|
int x2 = x + dx(d), y2 = y + dy(d);
|
|
if (x2 >= 0 && x2 < w && y2 >= 0 && y2 < h &&
|
|
soln[y2*w+x2] == TREE &&
|
|
!sc->links[y2*w+x2]) {
|
|
if (linkd)
|
|
break; /* found more than one */
|
|
else
|
|
linkd = d;
|
|
}
|
|
}
|
|
|
|
if (d == MAXDIR && linkd == 0) {
|
|
#ifdef SOLVER_DIAGNOSTICS
|
|
if (verbose)
|
|
printf("tent at %d,%d cannot link to anything\n",
|
|
x, y);
|
|
#endif
|
|
return 0; /* no solution exists */
|
|
} else if (d == MAXDIR) {
|
|
int x2 = x + dx(linkd), y2 = y + dy(linkd);
|
|
|
|
#ifdef SOLVER_DIAGNOSTICS
|
|
if (verbose)
|
|
printf("tent at %d,%d can only link to tree at"
|
|
" %d,%d\n", x, y, x2, y2);
|
|
#endif
|
|
|
|
sc->links[y*w+x] = linkd;
|
|
sc->links[y2*w+x2] = F(linkd);
|
|
done_something = TRUE;
|
|
}
|
|
}
|
|
|
|
if (done_something)
|
|
continue;
|
|
if (diff < 0)
|
|
break; /* don't do anything else! */
|
|
|
|
/*
|
|
* Mark a blank square as NONTENT if it is not orthogonally
|
|
* adjacent to any unmatched tree.
|
|
*/
|
|
for (y = 0; y < h; y++)
|
|
for (x = 0; x < w; x++)
|
|
if (soln[y*w+x] == BLANK) {
|
|
int can_be_tent = FALSE;
|
|
|
|
for (d = 1; d < MAXDIR; d++) {
|
|
int x2 = x + dx(d), y2 = y + dy(d);
|
|
if (x2 >= 0 && x2 < w && y2 >= 0 && y2 < h &&
|
|
soln[y2*w+x2] == TREE &&
|
|
!sc->links[y2*w+x2])
|
|
can_be_tent = TRUE;
|
|
}
|
|
|
|
if (!can_be_tent) {
|
|
#ifdef SOLVER_DIAGNOSTICS
|
|
if (verbose)
|
|
printf("%d,%d cannot be a tent (no adjacent"
|
|
" unmatched tree)\n", x, y);
|
|
#endif
|
|
soln[y*w+x] = NONTENT;
|
|
done_something = TRUE;
|
|
}
|
|
}
|
|
|
|
if (done_something)
|
|
continue;
|
|
|
|
/*
|
|
* Mark a blank square as NONTENT if it is (perhaps
|
|
* diagonally) adjacent to any other tent.
|
|
*/
|
|
for (y = 0; y < h; y++)
|
|
for (x = 0; x < w; x++)
|
|
if (soln[y*w+x] == BLANK) {
|
|
int dx, dy, imposs = FALSE;
|
|
|
|
for (dy = -1; dy <= +1; dy++)
|
|
for (dx = -1; dx <= +1; dx++)
|
|
if (dy || dx) {
|
|
int x2 = x + dx, y2 = y + dy;
|
|
if (x2 >= 0 && x2 < w && y2 >= 0 && y2 < h &&
|
|
soln[y2*w+x2] == TENT)
|
|
imposs = TRUE;
|
|
}
|
|
|
|
if (imposs) {
|
|
#ifdef SOLVER_DIAGNOSTICS
|
|
if (verbose)
|
|
printf("%d,%d cannot be a tent (adjacent tent)\n",
|
|
x, y);
|
|
#endif
|
|
soln[y*w+x] = NONTENT;
|
|
done_something = TRUE;
|
|
}
|
|
}
|
|
|
|
if (done_something)
|
|
continue;
|
|
|
|
/*
|
|
* Any tree which has exactly one {unattached tent, BLANK}
|
|
* adjacent to it must have its tent in that square.
|
|
*/
|
|
for (y = 0; y < h; y++)
|
|
for (x = 0; x < w; x++)
|
|
if (soln[y*w+x] == TREE && !sc->links[y*w+x]) {
|
|
int linkd = 0, linkd2 = 0, nd = 0;
|
|
|
|
for (d = 1; d < MAXDIR; d++) {
|
|
int x2 = x + dx(d), y2 = y + dy(d);
|
|
if (!(x2 >= 0 && x2 < w && y2 >= 0 && y2 < h))
|
|
continue;
|
|
if (soln[y2*w+x2] == BLANK ||
|
|
(soln[y2*w+x2] == TENT && !sc->links[y2*w+x2])) {
|
|
if (linkd)
|
|
linkd2 = d;
|
|
else
|
|
linkd = d;
|
|
nd++;
|
|
}
|
|
}
|
|
|
|
if (nd == 0) {
|
|
#ifdef SOLVER_DIAGNOSTICS
|
|
if (verbose)
|
|
printf("tree at %d,%d cannot link to anything\n",
|
|
x, y);
|
|
#endif
|
|
return 0; /* no solution exists */
|
|
} else if (nd == 1) {
|
|
int x2 = x + dx(linkd), y2 = y + dy(linkd);
|
|
|
|
#ifdef SOLVER_DIAGNOSTICS
|
|
if (verbose)
|
|
printf("tree at %d,%d can only link to tent at"
|
|
" %d,%d\n", x, y, x2, y2);
|
|
#endif
|
|
soln[y2*w+x2] = TENT;
|
|
sc->links[y*w+x] = linkd;
|
|
sc->links[y2*w+x2] = F(linkd);
|
|
done_something = TRUE;
|
|
} else if (nd == 2 && (!dx(linkd) != !dx(linkd2)) &&
|
|
diff >= DIFF_TRICKY) {
|
|
/*
|
|
* If there are two possible places where
|
|
* this tree's tent can go, and they are
|
|
* diagonally separated rather than being
|
|
* on opposite sides of the tree, then the
|
|
* square (other than the tree square)
|
|
* which is adjacent to both of them must
|
|
* be a non-tent.
|
|
*/
|
|
int x2 = x + dx(linkd) + dx(linkd2);
|
|
int y2 = y + dy(linkd) + dy(linkd2);
|
|
assert(x2 >= 0 && x2 < w && y2 >= 0 && y2 < h);
|
|
if (soln[y2*w+x2] == BLANK) {
|
|
#ifdef SOLVER_DIAGNOSTICS
|
|
if (verbose)
|
|
printf("possible tent locations for tree at"
|
|
" %d,%d rule out tent at %d,%d\n",
|
|
x, y, x2, y2);
|
|
#endif
|
|
soln[y2*w+x2] = NONTENT;
|
|
done_something = TRUE;
|
|
}
|
|
}
|
|
}
|
|
|
|
if (done_something)
|
|
continue;
|
|
|
|
/*
|
|
* If localised deductions about the trees and tents
|
|
* themselves haven't helped us, it's time to resort to the
|
|
* numbers round the grid edge. For each row and column, we
|
|
* go through all possible combinations of locations for
|
|
* the unplaced tents, rule out any which have adjacent
|
|
* tents, and spot any square which is given the same state
|
|
* by all remaining combinations.
|
|
*/
|
|
for (i = 0; i < w+h; i++) {
|
|
int start, step, len, start1, start2, n, k;
|
|
|
|
if (i < w) {
|
|
/*
|
|
* This is the number for a column.
|
|
*/
|
|
start = i;
|
|
step = w;
|
|
len = h;
|
|
if (i > 0)
|
|
start1 = start - 1;
|
|
else
|
|
start1 = -1;
|
|
if (i+1 < w)
|
|
start2 = start + 1;
|
|
else
|
|
start2 = -1;
|
|
} else {
|
|
/*
|
|
* This is the number for a row.
|
|
*/
|
|
start = (i-w)*w;
|
|
step = 1;
|
|
len = w;
|
|
if (i > w)
|
|
start1 = start - w;
|
|
else
|
|
start1 = -1;
|
|
if (i+1 < w+h)
|
|
start2 = start + w;
|
|
else
|
|
start2 = -1;
|
|
}
|
|
|
|
if (diff < DIFF_TRICKY) {
|
|
/*
|
|
* In Easy mode, we don't look at the effect of one
|
|
* row on the next (i.e. ruling out a square if all
|
|
* possibilities for an adjacent row place a tent
|
|
* next to it).
|
|
*/
|
|
start1 = start2 = -1;
|
|
}
|
|
|
|
k = numbers[i];
|
|
|
|
/*
|
|
* Count and store the locations of the free squares,
|
|
* and also count the number of tents already placed.
|
|
*/
|
|
n = 0;
|
|
for (j = 0; j < len; j++) {
|
|
if (soln[start+j*step] == TENT)
|
|
k--; /* one fewer tent to place */
|
|
else if (soln[start+j*step] == BLANK)
|
|
sc->locs[n++] = j;
|
|
}
|
|
|
|
if (n == 0)
|
|
continue; /* nothing left to do here */
|
|
|
|
/*
|
|
* Now we know we're placing k tents in n squares. Set
|
|
* up the first possibility.
|
|
*/
|
|
for (j = 0; j < n; j++)
|
|
sc->place[j] = (j < k ? TENT : NONTENT);
|
|
|
|
/*
|
|
* We're aiming to find squares in this row which are
|
|
* invariant over all valid possibilities. Thus, we
|
|
* maintain the current state of that invariance. We
|
|
* start everything off at MAGIC to indicate that it
|
|
* hasn't been set up yet.
|
|
*/
|
|
mrow = sc->mrows;
|
|
mrow1 = sc->mrows + len;
|
|
mrow2 = sc->mrows + 2*len;
|
|
trow = sc->trows;
|
|
trow1 = sc->trows + len;
|
|
trow2 = sc->trows + 2*len;
|
|
memset(mrow, MAGIC, 3*len);
|
|
|
|
/*
|
|
* And iterate over all possibilities.
|
|
*/
|
|
while (1) {
|
|
int p, valid;
|
|
|
|
/*
|
|
* See if this possibility is valid. The only way
|
|
* it can fail to be valid is if it contains two
|
|
* adjacent tents. (Other forms of invalidity, such
|
|
* as containing a tent adjacent to one already
|
|
* placed, will have been dealt with already by
|
|
* other parts of the solver.)
|
|
*/
|
|
valid = TRUE;
|
|
for (j = 0; j+1 < n; j++)
|
|
if (sc->place[j] == TENT &&
|
|
sc->place[j+1] == TENT &&
|
|
sc->locs[j+1] == sc->locs[j]+1) {
|
|
valid = FALSE;
|
|
break;
|
|
}
|
|
|
|
if (valid) {
|
|
/*
|
|
* Merge this valid combination into mrow.
|
|
*/
|
|
memset(trow, MAGIC, len);
|
|
memset(trow+len, BLANK, 2*len);
|
|
for (j = 0; j < n; j++) {
|
|
trow[sc->locs[j]] = sc->place[j];
|
|
if (sc->place[j] == TENT) {
|
|
int jj;
|
|
for (jj = sc->locs[j]-1; jj <= sc->locs[j]+1; jj++)
|
|
if (jj >= 0 && jj < len)
|
|
trow1[jj] = trow2[jj] = NONTENT;
|
|
}
|
|
}
|
|
|
|
for (j = 0; j < 3*len; j++) {
|
|
if (trow[j] == MAGIC)
|
|
continue;
|
|
if (mrow[j] == MAGIC || mrow[j] == trow[j]) {
|
|
/*
|
|
* Either this is the first valid
|
|
* placement we've found at all, or
|
|
* this square's contents are
|
|
* consistent with every previous valid
|
|
* combination.
|
|
*/
|
|
mrow[j] = trow[j];
|
|
} else {
|
|
/*
|
|
* This square's contents fail to match
|
|
* what they were in a different
|
|
* combination, so we cannot deduce
|
|
* anything about this square.
|
|
*/
|
|
mrow[j] = BLANK;
|
|
}
|
|
}
|
|
}
|
|
|
|
/*
|
|
* Find the next combination of k choices from n.
|
|
* We do this by finding the rightmost tent which
|
|
* can be moved one place right, doing so, and
|
|
* shunting all tents to the right of that as far
|
|
* left as they can go.
|
|
*/
|
|
p = 0;
|
|
for (j = n-1; j > 0; j--) {
|
|
if (sc->place[j] == TENT)
|
|
p++;
|
|
if (sc->place[j] == NONTENT && sc->place[j-1] == TENT) {
|
|
sc->place[j-1] = NONTENT;
|
|
sc->place[j] = TENT;
|
|
while (p--)
|
|
sc->place[++j] = TENT;
|
|
while (++j < n)
|
|
sc->place[j] = NONTENT;
|
|
break;
|
|
}
|
|
}
|
|
if (j <= 0)
|
|
break; /* we've finished */
|
|
}
|
|
|
|
/*
|
|
* It's just possible that _no_ placement was valid, in
|
|
* which case we have an internally inconsistent
|
|
* puzzle.
|
|
*/
|
|
if (mrow[sc->locs[0]] == MAGIC)
|
|
return 0; /* inconsistent */
|
|
|
|
/*
|
|
* Now go through mrow and see if there's anything
|
|
* we've deduced which wasn't already mentioned in soln.
|
|
*/
|
|
for (j = 0; j < len; j++) {
|
|
int whichrow;
|
|
|
|
for (whichrow = 0; whichrow < 3; whichrow++) {
|
|
char *mthis = mrow + whichrow * len;
|
|
int tstart = (whichrow == 0 ? start :
|
|
whichrow == 1 ? start1 : start2);
|
|
if (tstart >= 0 &&
|
|
mthis[j] != MAGIC && mthis[j] != BLANK &&
|
|
soln[tstart+j*step] == BLANK) {
|
|
int pos = tstart+j*step;
|
|
|
|
#ifdef SOLVER_DIAGNOSTICS
|
|
if (verbose)
|
|
printf("%s %d forces %s at %d,%d\n",
|
|
step==1 ? "row" : "column",
|
|
step==1 ? start/w : start,
|
|
mthis[j] == TENT ? "tent" : "non-tent",
|
|
pos % w, pos / w);
|
|
#endif
|
|
soln[pos] = mthis[j];
|
|
done_something = TRUE;
|
|
}
|
|
}
|
|
}
|
|
}
|
|
|
|
if (done_something)
|
|
continue;
|
|
|
|
if (!done_something)
|
|
break;
|
|
}
|
|
|
|
/*
|
|
* The solver has nothing further it can do. Return 1 if both
|
|
* soln and sc->links are completely filled in, or 2 otherwise.
|
|
*/
|
|
for (y = 0; y < h; y++)
|
|
for (x = 0; x < w; x++) {
|
|
if (soln[y*w+x] == BLANK)
|
|
return 2;
|
|
if (soln[y*w+x] != NONTENT && sc->links[y*w+x] == 0)
|
|
return 2;
|
|
}
|
|
|
|
return 1;
|
|
}
|
|
|
|
static char *new_game_desc(game_params *params, random_state *rs,
|
|
char **aux, int interactive)
|
|
{
|
|
int w = params->w, h = params->h;
|
|
int ntrees = w * h / 5;
|
|
char *grid = snewn(w*h, char);
|
|
char *puzzle = snewn(w*h, char);
|
|
int *numbers = snewn(w+h, int);
|
|
char *soln = snewn(w*h, char);
|
|
int *temp = snewn(2*w*h, int);
|
|
int maxedges = ntrees*4 + w*h;
|
|
int *edges = snewn(2*maxedges, int);
|
|
int *capacity = snewn(maxedges, int);
|
|
int *flow = snewn(maxedges, int);
|
|
struct solver_scratch *sc = new_scratch(w, h);
|
|
char *ret, *p;
|
|
int i, j, nedges;
|
|
|
|
/*
|
|
* Since this puzzle has many global deductions and doesn't
|
|
* permit limited clue sets, generating grids for this puzzle
|
|
* is hard enough that I see no better option than to simply
|
|
* generate a solution and see if it's unique and has the
|
|
* required difficulty. This turns out to be computationally
|
|
* plausible as well.
|
|
*
|
|
* We chose our tree count (hence also tent count) by dividing
|
|
* the total grid area by five above. Why five? Well, w*h/4 is
|
|
* the maximum number of tents you can _possibly_ fit into the
|
|
* grid without violating the separation criterion, and to
|
|
* achieve that you are constrained to a very small set of
|
|
* possible layouts (the obvious one with a tent at every
|
|
* (even,even) coordinate, and trivial variations thereon). So
|
|
* if we reduce the tent count a bit more, we enable more
|
|
* random-looking placement; 5 turns out to be a plausible
|
|
* figure which yields sensible puzzles. Increasing the tent
|
|
* count would give puzzles whose solutions were too regimented
|
|
* and could be solved by the use of that knowledge (and would
|
|
* also take longer to find a viable placement); decreasing it
|
|
* would make the grids emptier and more boring.
|
|
*
|
|
* Actually generating a grid is a matter of first placing the
|
|
* tents, and then placing the trees by the use of maxflow
|
|
* (finding a distinct square adjacent to every tent). We do it
|
|
* this way round because otherwise satisfying the tent
|
|
* separation condition would become onerous: most randomly
|
|
* chosen tent layouts do not satisfy this condition, so we'd
|
|
* have gone to a lot of work before finding that a candidate
|
|
* layout was unusable. Instead, we place the tents first and
|
|
* ensure they meet the separation criterion _before_ doing
|
|
* lots of computation; this works much better.
|
|
*
|
|
* The maxflow algorithm is not randomised, so employed naively
|
|
* it would give rise to grids with clear structure and
|
|
* directional bias. Hence, I assign the network nodes as seen
|
|
* by maxflow to be a _random_ permutation of the squares of
|
|
* the grid, so that any bias shown by maxflow towards
|
|
* low-numbered nodes is turned into a random bias.
|
|
*
|
|
* This generation strategy can fail at many points, including
|
|
* as early as tent placement (if you get a bad random order in
|
|
* which to greedily try the grid squares, you won't even
|
|
* manage to find enough mutually non-adjacent squares to put
|
|
* the tents in). Then it can fail if maxflow doesn't manage to
|
|
* find a good enough matching (i.e. the tent placements don't
|
|
* admit any adequate tree placements); and finally it can fail
|
|
* if the solver finds that the problem has the wrong
|
|
* difficulty (including being actually non-unique). All of
|
|
* these, however, are insufficiently frequent to cause
|
|
* trouble.
|
|
*/
|
|
|
|
if (params->diff > DIFF_EASY && params->w <= 4 && params->h <= 4)
|
|
params->diff = DIFF_EASY; /* downgrade to prevent tight loop */
|
|
|
|
while (1) {
|
|
/*
|
|
* Arrange the grid squares into a random order.
|
|
*/
|
|
for (i = 0; i < w*h; i++)
|
|
temp[i] = i;
|
|
shuffle(temp, w*h, sizeof(*temp), rs);
|
|
|
|
/*
|
|
* The first `ntrees' entries in temp which we can get
|
|
* without making two tents adjacent will be the tent
|
|
* locations.
|
|
*/
|
|
memset(grid, BLANK, w*h);
|
|
j = ntrees;
|
|
for (i = 0; i < w*h && j > 0; i++) {
|
|
int x = temp[i] % w, y = temp[i] / w;
|
|
int dy, dx, ok = TRUE;
|
|
|
|
for (dy = -1; dy <= +1; dy++)
|
|
for (dx = -1; dx <= +1; dx++)
|
|
if (x+dx >= 0 && x+dx < w &&
|
|
y+dy >= 0 && y+dy < h &&
|
|
grid[(y+dy)*w+(x+dx)] == TENT)
|
|
ok = FALSE;
|
|
|
|
if (ok) {
|
|
grid[temp[i]] = TENT;
|
|
j--;
|
|
}
|
|
}
|
|
if (j > 0)
|
|
continue; /* couldn't place all the tents */
|
|
|
|
/*
|
|
* Now we build up the list of graph edges.
|
|
*/
|
|
nedges = 0;
|
|
for (i = 0; i < w*h; i++) {
|
|
if (grid[temp[i]] == TENT) {
|
|
for (j = 0; j < w*h; j++) {
|
|
if (grid[temp[j]] != TENT) {
|
|
int xi = temp[i] % w, yi = temp[i] / w;
|
|
int xj = temp[j] % w, yj = temp[j] / w;
|
|
if (abs(xi-xj) + abs(yi-yj) == 1) {
|
|
edges[nedges*2] = i;
|
|
edges[nedges*2+1] = j;
|
|
capacity[nedges] = 1;
|
|
nedges++;
|
|
}
|
|
}
|
|
}
|
|
} else {
|
|
/*
|
|
* Special node w*h is the sink node; any non-tent node
|
|
* has an edge going to it.
|
|
*/
|
|
edges[nedges*2] = i;
|
|
edges[nedges*2+1] = w*h;
|
|
capacity[nedges] = 1;
|
|
nedges++;
|
|
}
|
|
}
|
|
|
|
/*
|
|
* Special node w*h+1 is the source node, with an edge going to
|
|
* every tent.
|
|
*/
|
|
for (i = 0; i < w*h; i++) {
|
|
if (grid[temp[i]] == TENT) {
|
|
edges[nedges*2] = w*h+1;
|
|
edges[nedges*2+1] = i;
|
|
capacity[nedges] = 1;
|
|
nedges++;
|
|
}
|
|
}
|
|
|
|
assert(nedges <= maxedges);
|
|
|
|
/*
|
|
* Now we're ready to call the maxflow algorithm to place the
|
|
* trees.
|
|
*/
|
|
j = maxflow(w*h+2, w*h+1, w*h, nedges, edges, capacity, flow, NULL);
|
|
|
|
if (j < ntrees)
|
|
continue; /* couldn't place all the tents */
|
|
|
|
/*
|
|
* We've placed the trees. Now we need to work out _where_
|
|
* we've placed them, which is a matter of reading back out
|
|
* from the `flow' array.
|
|
*/
|
|
for (i = 0; i < nedges; i++) {
|
|
if (edges[2*i] < w*h && edges[2*i+1] < w*h && flow[i] > 0)
|
|
grid[temp[edges[2*i+1]]] = TREE;
|
|
}
|
|
|
|
/*
|
|
* I think it looks ugly if there isn't at least one of
|
|
* _something_ (tent or tree) in each row and each column
|
|
* of the grid. This doesn't give any information away
|
|
* since a completely empty row/column is instantly obvious
|
|
* from the clues (it has no trees and a zero).
|
|
*/
|
|
for (i = 0; i < w; i++) {
|
|
for (j = 0; j < h; j++) {
|
|
if (grid[j*w+i] != BLANK)
|
|
break; /* found something in this column */
|
|
}
|
|
if (j == h)
|
|
break; /* found empty column */
|
|
}
|
|
if (i < w)
|
|
continue; /* a column was empty */
|
|
|
|
for (j = 0; j < h; j++) {
|
|
for (i = 0; i < w; i++) {
|
|
if (grid[j*w+i] != BLANK)
|
|
break; /* found something in this row */
|
|
}
|
|
if (i == w)
|
|
break; /* found empty row */
|
|
}
|
|
if (j < h)
|
|
continue; /* a row was empty */
|
|
|
|
/*
|
|
* Now set up the numbers round the edge.
|
|
*/
|
|
for (i = 0; i < w; i++) {
|
|
int n = 0;
|
|
for (j = 0; j < h; j++)
|
|
if (grid[j*w+i] == TENT)
|
|
n++;
|
|
numbers[i] = n;
|
|
}
|
|
for (i = 0; i < h; i++) {
|
|
int n = 0;
|
|
for (j = 0; j < w; j++)
|
|
if (grid[i*w+j] == TENT)
|
|
n++;
|
|
numbers[w+i] = n;
|
|
}
|
|
|
|
/*
|
|
* And now actually solve the puzzle, to see whether it's
|
|
* unique and has the required difficulty.
|
|
*/
|
|
for (i = 0; i < w*h; i++)
|
|
puzzle[i] = grid[i] == TREE ? TREE : BLANK;
|
|
i = tents_solve(w, h, puzzle, numbers, soln, sc, params->diff-1);
|
|
j = tents_solve(w, h, puzzle, numbers, soln, sc, params->diff);
|
|
|
|
/*
|
|
* We expect solving with difficulty params->diff to have
|
|
* succeeded (otherwise the problem is too hard), and
|
|
* solving with diff-1 to have failed (otherwise it's too
|
|
* easy).
|
|
*/
|
|
if (i == 2 && j == 1)
|
|
break;
|
|
}
|
|
|
|
/*
|
|
* That's it. Encode as a game ID.
|
|
*/
|
|
ret = snewn((w+h)*40 + ntrees + (w*h)/26 + 1, char);
|
|
p = ret;
|
|
j = 0;
|
|
for (i = 0; i <= w*h; i++) {
|
|
int c = (i < w*h ? grid[i] == TREE : 1);
|
|
if (c) {
|
|
*p++ = (j == 0 ? '_' : j-1 + 'a');
|
|
j = 0;
|
|
} else {
|
|
j++;
|
|
while (j > 25) {
|
|
*p++ = 'z';
|
|
j -= 25;
|
|
}
|
|
}
|
|
}
|
|
for (i = 0; i < w+h; i++)
|
|
p += sprintf(p, ",%d", numbers[i]);
|
|
*p++ = '\0';
|
|
ret = sresize(ret, p - ret, char);
|
|
|
|
/*
|
|
* And encode the solution as an aux_info.
|
|
*/
|
|
*aux = snewn(ntrees * 40, char);
|
|
p = *aux;
|
|
*p++ = 'S';
|
|
for (i = 0; i < w*h; i++)
|
|
if (grid[i] == TENT)
|
|
p += sprintf(p, ";T%d,%d", i%w, i/w);
|
|
*p++ = '\0';
|
|
*aux = sresize(*aux, p - *aux, char);
|
|
|
|
free_scratch(sc);
|
|
sfree(flow);
|
|
sfree(capacity);
|
|
sfree(edges);
|
|
sfree(temp);
|
|
sfree(soln);
|
|
sfree(numbers);
|
|
sfree(puzzle);
|
|
sfree(grid);
|
|
|
|
return ret;
|
|
}
|
|
|
|
static char *validate_desc(game_params *params, char *desc)
|
|
{
|
|
int w = params->w, h = params->h;
|
|
int area, i;
|
|
|
|
area = 0;
|
|
while (*desc && *desc != ',') {
|
|
if (*desc == '_')
|
|
area++;
|
|
else if (*desc >= 'a' && *desc < 'z')
|
|
area += *desc - 'a' + 2;
|
|
else if (*desc == 'z')
|
|
area += 25;
|
|
else if (*desc == '!' || *desc == '-')
|
|
/* do nothing */;
|
|
else
|
|
return "Invalid character in grid specification";
|
|
|
|
desc++;
|
|
}
|
|
|
|
for (i = 0; i < w+h; i++) {
|
|
if (!*desc)
|
|
return "Not enough numbers given after grid specification";
|
|
else if (*desc != ',')
|
|
return "Invalid character in number list";
|
|
desc++;
|
|
while (*desc && isdigit((unsigned char)*desc)) desc++;
|
|
}
|
|
|
|
if (*desc)
|
|
return "Unexpected additional data at end of game description";
|
|
return NULL;
|
|
}
|
|
|
|
static game_state *new_game(midend *me, game_params *params, char *desc)
|
|
{
|
|
int w = params->w, h = params->h;
|
|
game_state *state = snew(game_state);
|
|
int i;
|
|
|
|
state->p = *params; /* structure copy */
|
|
state->grid = snewn(w*h, char);
|
|
state->numbers = snew(struct numbers);
|
|
state->numbers->refcount = 1;
|
|
state->numbers->numbers = snewn(w+h, int);
|
|
state->completed = state->used_solve = FALSE;
|
|
|
|
i = 0;
|
|
memset(state->grid, BLANK, w*h);
|
|
|
|
while (*desc) {
|
|
int run, type;
|
|
|
|
type = TREE;
|
|
|
|
if (*desc == '_')
|
|
run = 0;
|
|
else if (*desc >= 'a' && *desc < 'z')
|
|
run = *desc - ('a'-1);
|
|
else if (*desc == 'z') {
|
|
run = 25;
|
|
type = BLANK;
|
|
} else {
|
|
assert(*desc == '!' || *desc == '-');
|
|
run = -1;
|
|
type = (*desc == '!' ? TENT : NONTENT);
|
|
}
|
|
|
|
desc++;
|
|
|
|
i += run;
|
|
assert(i >= 0 && i <= w*h);
|
|
if (i == w*h) {
|
|
assert(type == TREE);
|
|
break;
|
|
} else {
|
|
if (type != BLANK)
|
|
state->grid[i++] = type;
|
|
}
|
|
}
|
|
|
|
for (i = 0; i < w+h; i++) {
|
|
assert(*desc == ',');
|
|
desc++;
|
|
state->numbers->numbers[i] = atoi(desc);
|
|
while (*desc && isdigit((unsigned char)*desc)) desc++;
|
|
}
|
|
|
|
assert(!*desc);
|
|
|
|
return state;
|
|
}
|
|
|
|
static game_state *dup_game(game_state *state)
|
|
{
|
|
int w = state->p.w, h = state->p.h;
|
|
game_state *ret = snew(game_state);
|
|
|
|
ret->p = state->p; /* structure copy */
|
|
ret->grid = snewn(w*h, char);
|
|
memcpy(ret->grid, state->grid, w*h);
|
|
ret->numbers = state->numbers;
|
|
state->numbers->refcount++;
|
|
ret->completed = state->completed;
|
|
ret->used_solve = state->used_solve;
|
|
|
|
return ret;
|
|
}
|
|
|
|
static void free_game(game_state *state)
|
|
{
|
|
if (--state->numbers->refcount <= 0) {
|
|
sfree(state->numbers->numbers);
|
|
sfree(state->numbers);
|
|
}
|
|
sfree(state->grid);
|
|
sfree(state);
|
|
}
|
|
|
|
static char *solve_game(game_state *state, game_state *currstate,
|
|
char *aux, char **error)
|
|
{
|
|
int w = state->p.w, h = state->p.h;
|
|
|
|
if (aux) {
|
|
/*
|
|
* If we already have the solution, save ourselves some
|
|
* time.
|
|
*/
|
|
return dupstr(aux);
|
|
} else {
|
|
struct solver_scratch *sc = new_scratch(w, h);
|
|
char *soln;
|
|
int ret;
|
|
char *move, *p;
|
|
int i;
|
|
|
|
soln = snewn(w*h, char);
|
|
ret = tents_solve(w, h, state->grid, state->numbers->numbers,
|
|
soln, sc, DIFFCOUNT-1);
|
|
free_scratch(sc);
|
|
if (ret != 1) {
|
|
sfree(soln);
|
|
if (ret == 0)
|
|
*error = "This puzzle is not self-consistent";
|
|
else
|
|
*error = "Unable to find a unique solution for this puzzle";
|
|
return NULL;
|
|
}
|
|
|
|
/*
|
|
* Construct a move string which turns the current state
|
|
* into the solved state.
|
|
*/
|
|
move = snewn(w*h * 40, char);
|
|
p = move;
|
|
*p++ = 'S';
|
|
for (i = 0; i < w*h; i++)
|
|
if (soln[i] == TENT)
|
|
p += sprintf(p, ";T%d,%d", i%w, i/w);
|
|
*p++ = '\0';
|
|
move = sresize(move, p - move, char);
|
|
|
|
sfree(soln);
|
|
|
|
return move;
|
|
}
|
|
}
|
|
|
|
static char *game_text_format(game_state *state)
|
|
{
|
|
int w = state->p.w, h = state->p.h;
|
|
char *ret, *p;
|
|
int x, y;
|
|
|
|
/*
|
|
* FIXME: We currently do not print the numbers round the edges
|
|
* of the grid. I need to work out a sensible way of doing this
|
|
* even when the column numbers exceed 9.
|
|
*
|
|
* In the absence of those numbers, the result size is h lines
|
|
* of w+1 characters each, plus a NUL.
|
|
*
|
|
* This function is currently only used by the standalone
|
|
* solver; until I make it look more sensible, I won't enable
|
|
* it in the main game structure.
|
|
*/
|
|
ret = snewn(h*(w+1) + 1, char);
|
|
p = ret;
|
|
for (y = 0; y < h; y++) {
|
|
for (x = 0; x < w; x++) {
|
|
*p = (state->grid[y*w+x] == BLANK ? '.' :
|
|
state->grid[y*w+x] == TREE ? 'T' :
|
|
state->grid[y*w+x] == TENT ? '*' :
|
|
state->grid[y*w+x] == NONTENT ? '-' : '?');
|
|
p++;
|
|
}
|
|
*p++ = '\n';
|
|
}
|
|
*p++ = '\0';
|
|
|
|
return ret;
|
|
}
|
|
|
|
struct game_ui {
|
|
int dsx, dsy; /* coords of drag start */
|
|
int dex, dey; /* coords of drag end */
|
|
int drag_button; /* -1 for none, or a button code */
|
|
int drag_ok; /* dragged off the window, to cancel */
|
|
};
|
|
|
|
static game_ui *new_ui(game_state *state)
|
|
{
|
|
game_ui *ui = snew(game_ui);
|
|
ui->dsx = ui->dsy = -1;
|
|
ui->dex = ui->dey = -1;
|
|
ui->drag_button = -1;
|
|
ui->drag_ok = FALSE;
|
|
return ui;
|
|
}
|
|
|
|
static void free_ui(game_ui *ui)
|
|
{
|
|
sfree(ui);
|
|
}
|
|
|
|
static char *encode_ui(game_ui *ui)
|
|
{
|
|
return NULL;
|
|
}
|
|
|
|
static void decode_ui(game_ui *ui, char *encoding)
|
|
{
|
|
}
|
|
|
|
static void game_changed_state(game_ui *ui, game_state *oldstate,
|
|
game_state *newstate)
|
|
{
|
|
}
|
|
|
|
struct game_drawstate {
|
|
int tilesize;
|
|
int started;
|
|
game_params p;
|
|
char *drawn;
|
|
};
|
|
|
|
#define PREFERRED_TILESIZE 32
|
|
#define TILESIZE (ds->tilesize)
|
|
#define TLBORDER (TILESIZE/2)
|
|
#define BRBORDER (TILESIZE*3/2)
|
|
#define COORD(x) ( (x) * TILESIZE + TLBORDER )
|
|
#define FROMCOORD(x) ( ((x) - TLBORDER + TILESIZE) / TILESIZE - 1 )
|
|
|
|
#define FLASH_TIME 0.30F
|
|
|
|
static int drag_xform(game_ui *ui, int x, int y, int v)
|
|
{
|
|
int xmin, ymin, xmax, ymax;
|
|
|
|
xmin = min(ui->dsx, ui->dex);
|
|
xmax = max(ui->dsx, ui->dex);
|
|
ymin = min(ui->dsy, ui->dey);
|
|
ymax = max(ui->dsy, ui->dey);
|
|
|
|
/*
|
|
* Left-dragging has no effect, so we treat a left-drag as a
|
|
* single click on dsx,dsy.
|
|
*/
|
|
if (ui->drag_button == LEFT_BUTTON) {
|
|
xmin = xmax = ui->dsx;
|
|
ymin = ymax = ui->dsy;
|
|
}
|
|
|
|
if (x < xmin || x > xmax || y < ymin || y > ymax)
|
|
return v; /* no change outside drag area */
|
|
|
|
if (v == TREE)
|
|
return v; /* trees are inviolate always */
|
|
|
|
if (xmin == xmax && ymin == ymax) {
|
|
/*
|
|
* Results of a simple click. Left button sets blanks to
|
|
* tents; right button sets blanks to non-tents; either
|
|
* button clears a non-blank square.
|
|
*/
|
|
if (ui->drag_button == LEFT_BUTTON)
|
|
v = (v == BLANK ? TENT : BLANK);
|
|
else
|
|
v = (v == BLANK ? NONTENT : BLANK);
|
|
} else {
|
|
/*
|
|
* Results of a drag. Left-dragging has no effect.
|
|
* Right-dragging sets all blank squares to non-tents and
|
|
* has no effect on anything else.
|
|
*/
|
|
if (ui->drag_button == RIGHT_BUTTON)
|
|
v = (v == BLANK ? NONTENT : v);
|
|
else
|
|
/* do nothing */;
|
|
}
|
|
|
|
return v;
|
|
}
|
|
|
|
static char *interpret_move(game_state *state, game_ui *ui, game_drawstate *ds,
|
|
int x, int y, int button)
|
|
{
|
|
int w = state->p.w, h = state->p.h;
|
|
|
|
if (button == LEFT_BUTTON || button == RIGHT_BUTTON) {
|
|
x = FROMCOORD(x);
|
|
y = FROMCOORD(y);
|
|
if (x < 0 || y < 0 || x >= w || y >= h)
|
|
return NULL;
|
|
|
|
ui->drag_button = button;
|
|
ui->dsx = ui->dex = x;
|
|
ui->dsy = ui->dey = y;
|
|
ui->drag_ok = TRUE;
|
|
return ""; /* ui updated */
|
|
}
|
|
|
|
if ((IS_MOUSE_DRAG(button) || IS_MOUSE_RELEASE(button)) &&
|
|
ui->drag_button > 0) {
|
|
int xmin, ymin, xmax, ymax;
|
|
char *buf, *sep, tmpbuf[80];
|
|
int buflen, bufsize, tmplen;
|
|
|
|
x = FROMCOORD(x);
|
|
y = FROMCOORD(y);
|
|
if (x < 0 || y < 0 || x >= w || y >= h) {
|
|
ui->drag_ok = FALSE;
|
|
} else {
|
|
/*
|
|
* Drags are limited to one row or column. Hence, we
|
|
* work out which coordinate is closer to the drag
|
|
* start, and move it _to_ the drag start.
|
|
*/
|
|
if (abs(x - ui->dsx) < abs(y - ui->dsy))
|
|
x = ui->dsx;
|
|
else
|
|
y = ui->dsy;
|
|
|
|
ui->dex = x;
|
|
ui->dey = y;
|
|
|
|
ui->drag_ok = TRUE;
|
|
}
|
|
|
|
if (IS_MOUSE_DRAG(button))
|
|
return ""; /* ui updated */
|
|
|
|
/*
|
|
* The drag has been released. Enact it.
|
|
*/
|
|
if (!ui->drag_ok) {
|
|
ui->drag_button = -1;
|
|
return ""; /* drag was just cancelled */
|
|
}
|
|
|
|
xmin = min(ui->dsx, ui->dex);
|
|
xmax = max(ui->dsx, ui->dex);
|
|
ymin = min(ui->dsy, ui->dey);
|
|
ymax = max(ui->dsy, ui->dey);
|
|
assert(0 <= xmin && xmin <= xmax && xmax < w);
|
|
assert(0 <= ymin && ymin <= ymax && ymax < h);
|
|
|
|
buflen = 0;
|
|
bufsize = 256;
|
|
buf = snewn(bufsize, char);
|
|
sep = "";
|
|
for (y = ymin; y <= ymax; y++)
|
|
for (x = xmin; x <= xmax; x++) {
|
|
int v = drag_xform(ui, x, y, state->grid[y*w+x]);
|
|
if (state->grid[y*w+x] != v) {
|
|
tmplen = sprintf(tmpbuf, "%s%c%d,%d", sep,
|
|
(int)(v == BLANK ? 'B' :
|
|
v == TENT ? 'T' : 'N'),
|
|
x, y);
|
|
sep = ";";
|
|
|
|
if (buflen + tmplen >= bufsize) {
|
|
bufsize = buflen + tmplen + 256;
|
|
buf = sresize(buf, bufsize, char);
|
|
}
|
|
|
|
strcpy(buf+buflen, tmpbuf);
|
|
buflen += tmplen;
|
|
}
|
|
}
|
|
|
|
ui->drag_button = -1; /* drag is terminated */
|
|
|
|
if (buflen == 0) {
|
|
sfree(buf);
|
|
return ""; /* ui updated (drag was terminated) */
|
|
} else {
|
|
buf[buflen] = '\0';
|
|
return buf;
|
|
}
|
|
}
|
|
|
|
return NULL;
|
|
}
|
|
|
|
static game_state *execute_move(game_state *state, char *move)
|
|
{
|
|
int w = state->p.w, h = state->p.h;
|
|
char c;
|
|
int x, y, m, n, i, j;
|
|
game_state *ret = dup_game(state);
|
|
|
|
while (*move) {
|
|
c = *move;
|
|
if (c == 'S') {
|
|
int i;
|
|
ret->used_solve = TRUE;
|
|
/*
|
|
* Set all non-tree squares to NONTENT. The rest of the
|
|
* solve move will fill the tents in over the top.
|
|
*/
|
|
for (i = 0; i < w*h; i++)
|
|
if (ret->grid[i] != TREE)
|
|
ret->grid[i] = NONTENT;
|
|
move++;
|
|
} else if (c == 'B' || c == 'T' || c == 'N') {
|
|
move++;
|
|
if (sscanf(move, "%d,%d%n", &x, &y, &n) != 2 ||
|
|
x < 0 || y < 0 || x >= w || y >= h) {
|
|
free_game(ret);
|
|
return NULL;
|
|
}
|
|
if (ret->grid[y*w+x] == TREE) {
|
|
free_game(ret);
|
|
return NULL;
|
|
}
|
|
ret->grid[y*w+x] = (c == 'B' ? BLANK : c == 'T' ? TENT : NONTENT);
|
|
move += n;
|
|
} else {
|
|
free_game(ret);
|
|
return NULL;
|
|
}
|
|
if (*move == ';')
|
|
move++;
|
|
else if (*move) {
|
|
free_game(ret);
|
|
return NULL;
|
|
}
|
|
}
|
|
|
|
/*
|
|
* Check for completion.
|
|
*/
|
|
for (i = n = m = 0; i < w*h; i++) {
|
|
if (ret->grid[i] == TENT)
|
|
n++;
|
|
else if (ret->grid[i] == TREE)
|
|
m++;
|
|
}
|
|
if (n == m) {
|
|
int nedges, maxedges, *edges, *capacity, *flow;
|
|
|
|
/*
|
|
* We have the right number of tents, which is a
|
|
* precondition for the game being complete. Now check that
|
|
* the numbers add up.
|
|
*/
|
|
for (i = 0; i < w; i++) {
|
|
n = 0;
|
|
for (j = 0; j < h; j++)
|
|
if (ret->grid[j*w+i] == TENT)
|
|
n++;
|
|
if (ret->numbers->numbers[i] != n)
|
|
goto completion_check_done;
|
|
}
|
|
for (i = 0; i < h; i++) {
|
|
n = 0;
|
|
for (j = 0; j < w; j++)
|
|
if (ret->grid[i*w+j] == TENT)
|
|
n++;
|
|
if (ret->numbers->numbers[w+i] != n)
|
|
goto completion_check_done;
|
|
}
|
|
/*
|
|
* Also, check that no two tents are adjacent.
|
|
*/
|
|
for (y = 0; y < h; y++)
|
|
for (x = 0; x < w; x++) {
|
|
if (x+1 < w &&
|
|
ret->grid[y*w+x] == TENT && ret->grid[y*w+x+1] == TENT)
|
|
goto completion_check_done;
|
|
if (y+1 < h &&
|
|
ret->grid[y*w+x] == TENT && ret->grid[(y+1)*w+x] == TENT)
|
|
goto completion_check_done;
|
|
if (x+1 < w && y+1 < h) {
|
|
if (ret->grid[y*w+x] == TENT &&
|
|
ret->grid[(y+1)*w+(x+1)] == TENT)
|
|
goto completion_check_done;
|
|
if (ret->grid[(y+1)*w+x] == TENT &&
|
|
ret->grid[y*w+(x+1)] == TENT)
|
|
goto completion_check_done;
|
|
}
|
|
}
|
|
|
|
/*
|
|
* OK; we have the right number of tents, they match the
|
|
* numeric clues, and they satisfy the non-adjacency
|
|
* criterion. Finally, we need to verify that they can be
|
|
* placed in a one-to-one matching with the trees such that
|
|
* every tent is orthogonally adjacent to its tree.
|
|
*
|
|
* This bit is where the hard work comes in: we have to do
|
|
* it by finding such a matching using maxflow.
|
|
*
|
|
* So we construct a network with one special source node,
|
|
* one special sink node, one node per tent, and one node
|
|
* per tree.
|
|
*/
|
|
maxedges = 6 * m;
|
|
edges = snewn(2 * maxedges, int);
|
|
capacity = snewn(maxedges, int);
|
|
flow = snewn(maxedges, int);
|
|
nedges = 0;
|
|
/*
|
|
* Node numbering:
|
|
*
|
|
* 0..w*h trees/tents
|
|
* w*h source
|
|
* w*h+1 sink
|
|
*/
|
|
for (y = 0; y < h; y++)
|
|
for (x = 0; x < w; x++)
|
|
if (ret->grid[y*w+x] == TREE) {
|
|
int d;
|
|
|
|
/*
|
|
* Here we use the direction enum declared for
|
|
* the solver. We make use of the fact that the
|
|
* directions are declared in the order
|
|
* U,L,R,D, meaning that we go through the four
|
|
* neighbours of any square in numerically
|
|
* increasing order.
|
|
*/
|
|
for (d = 1; d < MAXDIR; d++) {
|
|
int x2 = x + dx(d), y2 = y + dy(d);
|
|
if (x2 >= 0 && x2 < w && y2 >= 0 && y2 < h &&
|
|
ret->grid[y2*w+x2] == TENT) {
|
|
assert(nedges < maxedges);
|
|
edges[nedges*2] = y*w+x;
|
|
edges[nedges*2+1] = y2*w+x2;
|
|
capacity[nedges] = 1;
|
|
nedges++;
|
|
}
|
|
}
|
|
} else if (ret->grid[y*w+x] == TENT) {
|
|
assert(nedges < maxedges);
|
|
edges[nedges*2] = y*w+x;
|
|
edges[nedges*2+1] = w*h+1; /* edge going to sink */
|
|
capacity[nedges] = 1;
|
|
nedges++;
|
|
}
|
|
for (y = 0; y < h; y++)
|
|
for (x = 0; x < w; x++)
|
|
if (ret->grid[y*w+x] == TREE) {
|
|
assert(nedges < maxedges);
|
|
edges[nedges*2] = w*h; /* edge coming from source */
|
|
edges[nedges*2+1] = y*w+x;
|
|
capacity[nedges] = 1;
|
|
nedges++;
|
|
}
|
|
n = maxflow(w*h+2, w*h, w*h+1, nedges, edges, capacity, flow, NULL);
|
|
|
|
sfree(flow);
|
|
sfree(capacity);
|
|
sfree(edges);
|
|
|
|
if (n != m)
|
|
goto completion_check_done;
|
|
|
|
/*
|
|
* We haven't managed to fault the grid on any count. Score!
|
|
*/
|
|
ret->completed = TRUE;
|
|
}
|
|
completion_check_done:
|
|
|
|
return ret;
|
|
}
|
|
|
|
/* ----------------------------------------------------------------------
|
|
* Drawing routines.
|
|
*/
|
|
|
|
static void game_compute_size(game_params *params, int tilesize,
|
|
int *x, int *y)
|
|
{
|
|
/* fool the macros */
|
|
struct dummy { int tilesize; } dummy = { tilesize }, *ds = &dummy;
|
|
|
|
*x = TLBORDER + BRBORDER + TILESIZE * params->w;
|
|
*y = TLBORDER + BRBORDER + TILESIZE * params->h;
|
|
}
|
|
|
|
static void game_set_size(drawing *dr, game_drawstate *ds,
|
|
game_params *params, int tilesize)
|
|
{
|
|
ds->tilesize = tilesize;
|
|
}
|
|
|
|
static float *game_colours(frontend *fe, int *ncolours)
|
|
{
|
|
float *ret = snewn(3 * NCOLOURS, float);
|
|
|
|
frontend_default_colour(fe, &ret[COL_BACKGROUND * 3]);
|
|
|
|
ret[COL_GRID * 3 + 0] = 0.0F;
|
|
ret[COL_GRID * 3 + 1] = 0.0F;
|
|
ret[COL_GRID * 3 + 2] = 0.0F;
|
|
|
|
ret[COL_GRASS * 3 + 0] = 0.7F;
|
|
ret[COL_GRASS * 3 + 1] = 1.0F;
|
|
ret[COL_GRASS * 3 + 2] = 0.5F;
|
|
|
|
ret[COL_TREETRUNK * 3 + 0] = 0.6F;
|
|
ret[COL_TREETRUNK * 3 + 1] = 0.4F;
|
|
ret[COL_TREETRUNK * 3 + 2] = 0.0F;
|
|
|
|
ret[COL_TREELEAF * 3 + 0] = 0.0F;
|
|
ret[COL_TREELEAF * 3 + 1] = 0.7F;
|
|
ret[COL_TREELEAF * 3 + 2] = 0.0F;
|
|
|
|
ret[COL_TENT * 3 + 0] = 0.8F;
|
|
ret[COL_TENT * 3 + 1] = 0.7F;
|
|
ret[COL_TENT * 3 + 2] = 0.0F;
|
|
|
|
*ncolours = NCOLOURS;
|
|
return ret;
|
|
}
|
|
|
|
static game_drawstate *game_new_drawstate(drawing *dr, game_state *state)
|
|
{
|
|
int w = state->p.w, h = state->p.h;
|
|
struct game_drawstate *ds = snew(struct game_drawstate);
|
|
|
|
ds->tilesize = 0;
|
|
ds->started = FALSE;
|
|
ds->p = state->p; /* structure copy */
|
|
ds->drawn = snewn(w*h, char);
|
|
memset(ds->drawn, MAGIC, w*h);
|
|
|
|
return ds;
|
|
}
|
|
|
|
static void game_free_drawstate(drawing *dr, game_drawstate *ds)
|
|
{
|
|
sfree(ds->drawn);
|
|
sfree(ds);
|
|
}
|
|
|
|
static void draw_tile(drawing *dr, game_drawstate *ds,
|
|
int x, int y, int v, int printing)
|
|
{
|
|
int tx = COORD(x), ty = COORD(y);
|
|
int cx = tx + TILESIZE/2, cy = ty + TILESIZE/2;
|
|
|
|
clip(dr, tx+1, ty+1, TILESIZE-1, TILESIZE-1);
|
|
|
|
if (!printing)
|
|
draw_rect(dr, tx+1, ty+1, TILESIZE-1, TILESIZE-1,
|
|
(v == BLANK ? COL_BACKGROUND : COL_GRASS));
|
|
|
|
if (v == TREE) {
|
|
int i;
|
|
|
|
(printing ? draw_rect_outline : draw_rect)
|
|
(dr, cx-TILESIZE/15, ty+TILESIZE*3/10,
|
|
2*(TILESIZE/15)+1, (TILESIZE*9/10 - TILESIZE*3/10),
|
|
COL_TREETRUNK);
|
|
|
|
for (i = 0; i < (printing ? 2 : 1); i++) {
|
|
int col = (i == 1 ? COL_BACKGROUND : COL_TREELEAF);
|
|
int sub = i * (TILESIZE/32);
|
|
draw_circle(dr, cx, ty+TILESIZE*4/10, TILESIZE/4 - sub,
|
|
col, col);
|
|
draw_circle(dr, cx+TILESIZE/5, ty+TILESIZE/4, TILESIZE/8 - sub,
|
|
col, col);
|
|
draw_circle(dr, cx-TILESIZE/5, ty+TILESIZE/4, TILESIZE/8 - sub,
|
|
col, col);
|
|
draw_circle(dr, cx+TILESIZE/4, ty+TILESIZE*6/13, TILESIZE/8 - sub,
|
|
col, col);
|
|
draw_circle(dr, cx-TILESIZE/4, ty+TILESIZE*6/13, TILESIZE/8 - sub,
|
|
col, col);
|
|
}
|
|
} else if (v == TENT) {
|
|
int coords[6];
|
|
coords[0] = cx - TILESIZE/3;
|
|
coords[1] = cy + TILESIZE/3;
|
|
coords[2] = cx + TILESIZE/3;
|
|
coords[3] = cy + TILESIZE/3;
|
|
coords[4] = cx;
|
|
coords[5] = cy - TILESIZE/3;
|
|
draw_polygon(dr, coords, 3, (printing ? -1 : COL_TENT), COL_TENT);
|
|
}
|
|
|
|
unclip(dr);
|
|
draw_update(dr, tx+1, ty+1, TILESIZE-1, TILESIZE-1);
|
|
}
|
|
|
|
/*
|
|
* Internal redraw function, used for printing as well as drawing.
|
|
*/
|
|
static void int_redraw(drawing *dr, game_drawstate *ds, game_state *oldstate,
|
|
game_state *state, int dir, game_ui *ui,
|
|
float animtime, float flashtime, int printing)
|
|
{
|
|
int w = state->p.w, h = state->p.h;
|
|
int x, y, flashing;
|
|
|
|
if (printing || !ds->started) {
|
|
if (!printing) {
|
|
int ww, wh;
|
|
game_compute_size(&state->p, TILESIZE, &ww, &wh);
|
|
draw_rect(dr, 0, 0, ww, wh, COL_BACKGROUND);
|
|
draw_update(dr, 0, 0, ww, wh);
|
|
ds->started = TRUE;
|
|
}
|
|
|
|
if (printing)
|
|
print_line_width(dr, TILESIZE/64);
|
|
|
|
/*
|
|
* Draw the grid.
|
|
*/
|
|
for (y = 0; y <= h; y++)
|
|
draw_line(dr, COORD(0), COORD(y), COORD(w), COORD(y), COL_GRID);
|
|
for (x = 0; x <= w; x++)
|
|
draw_line(dr, COORD(x), COORD(0), COORD(x), COORD(h), COL_GRID);
|
|
|
|
/*
|
|
* Draw the numbers.
|
|
*/
|
|
for (y = 0; y < h; y++) {
|
|
char buf[80];
|
|
sprintf(buf, "%d", state->numbers->numbers[y+w]);
|
|
draw_text(dr, COORD(w+1), COORD(y) + TILESIZE/2,
|
|
FONT_VARIABLE, TILESIZE/2, ALIGN_HRIGHT|ALIGN_VCENTRE,
|
|
COL_GRID, buf);
|
|
}
|
|
for (x = 0; x < w; x++) {
|
|
char buf[80];
|
|
sprintf(buf, "%d", state->numbers->numbers[x]);
|
|
draw_text(dr, COORD(x) + TILESIZE/2, COORD(h+1),
|
|
FONT_VARIABLE, TILESIZE/2, ALIGN_HCENTRE|ALIGN_VNORMAL,
|
|
COL_GRID, buf);
|
|
}
|
|
}
|
|
|
|
if (flashtime > 0)
|
|
flashing = (int)(flashtime * 3 / FLASH_TIME) != 1;
|
|
else
|
|
flashing = FALSE;
|
|
|
|
/*
|
|
* Draw the grid.
|
|
*/
|
|
for (y = 0; y < h; y++)
|
|
for (x = 0; x < w; x++) {
|
|
int v = state->grid[y*w+x];
|
|
|
|
/*
|
|
* We deliberately do not take drag_ok into account
|
|
* here, because user feedback suggests that it's
|
|
* marginally nicer not to have the drag effects
|
|
* flickering on and off disconcertingly.
|
|
*/
|
|
if (ui && ui->drag_button >= 0)
|
|
v = drag_xform(ui, x, y, v);
|
|
|
|
if (flashing && (v == TREE || v == TENT))
|
|
v = NONTENT;
|
|
|
|
if (printing || ds->drawn[y*w+x] != v) {
|
|
draw_tile(dr, ds, x, y, v, printing);
|
|
if (!printing)
|
|
ds->drawn[y*w+x] = v;
|
|
}
|
|
}
|
|
}
|
|
|
|
static void game_redraw(drawing *dr, game_drawstate *ds, game_state *oldstate,
|
|
game_state *state, int dir, game_ui *ui,
|
|
float animtime, float flashtime)
|
|
{
|
|
int_redraw(dr, ds, oldstate, state, dir, ui, animtime, flashtime, FALSE);
|
|
}
|
|
|
|
static float game_anim_length(game_state *oldstate, game_state *newstate,
|
|
int dir, game_ui *ui)
|
|
{
|
|
return 0.0F;
|
|
}
|
|
|
|
static float game_flash_length(game_state *oldstate, game_state *newstate,
|
|
int dir, game_ui *ui)
|
|
{
|
|
if (!oldstate->completed && newstate->completed &&
|
|
!oldstate->used_solve && !newstate->used_solve)
|
|
return FLASH_TIME;
|
|
|
|
return 0.0F;
|
|
}
|
|
|
|
static int game_timing_state(game_state *state, game_ui *ui)
|
|
{
|
|
return TRUE;
|
|
}
|
|
|
|
static void game_print_size(game_params *params, float *x, float *y)
|
|
{
|
|
int pw, ph;
|
|
|
|
/*
|
|
* I'll use 6mm squares by default.
|
|
*/
|
|
game_compute_size(params, 600, &pw, &ph);
|
|
*x = pw / 100.0;
|
|
*y = ph / 100.0;
|
|
}
|
|
|
|
static void game_print(drawing *dr, game_state *state, int tilesize)
|
|
{
|
|
int c;
|
|
|
|
/* Ick: fake up `ds->tilesize' for macro expansion purposes */
|
|
game_drawstate ads, *ds = &ads;
|
|
game_set_size(dr, ds, NULL, tilesize);
|
|
|
|
c = print_mono_colour(dr, 1); assert(c == COL_BACKGROUND);
|
|
c = print_mono_colour(dr, 0); assert(c == COL_GRID);
|
|
c = print_mono_colour(dr, 1); assert(c == COL_GRASS);
|
|
c = print_mono_colour(dr, 0); assert(c == COL_TREETRUNK);
|
|
c = print_mono_colour(dr, 0); assert(c == COL_TREELEAF);
|
|
c = print_mono_colour(dr, 0); assert(c == COL_TENT);
|
|
|
|
int_redraw(dr, ds, NULL, state, +1, NULL, 0.0F, 0.0F, TRUE);
|
|
}
|
|
|
|
#ifdef COMBINED
|
|
#define thegame tents
|
|
#endif
|
|
|
|
const struct game thegame = {
|
|
"Tents", "games.tents", "tents",
|
|
default_params,
|
|
game_fetch_preset,
|
|
decode_params,
|
|
encode_params,
|
|
free_params,
|
|
dup_params,
|
|
TRUE, game_configure, custom_params,
|
|
validate_params,
|
|
new_game_desc,
|
|
validate_desc,
|
|
new_game,
|
|
dup_game,
|
|
free_game,
|
|
TRUE, solve_game,
|
|
FALSE, game_text_format,
|
|
new_ui,
|
|
free_ui,
|
|
encode_ui,
|
|
decode_ui,
|
|
game_changed_state,
|
|
interpret_move,
|
|
execute_move,
|
|
PREFERRED_TILESIZE, game_compute_size, game_set_size,
|
|
game_colours,
|
|
game_new_drawstate,
|
|
game_free_drawstate,
|
|
game_redraw,
|
|
game_anim_length,
|
|
game_flash_length,
|
|
TRUE, FALSE, game_print_size, game_print,
|
|
FALSE, /* wants_statusbar */
|
|
FALSE, game_timing_state,
|
|
REQUIRE_RBUTTON, /* flags */
|
|
};
|
|
|
|
#ifdef STANDALONE_SOLVER
|
|
|
|
#include <stdarg.h>
|
|
|
|
int main(int argc, char **argv)
|
|
{
|
|
game_params *p;
|
|
game_state *s, *s2;
|
|
char *id = NULL, *desc, *err;
|
|
int grade = FALSE;
|
|
int ret, diff, really_verbose = FALSE;
|
|
struct solver_scratch *sc;
|
|
|
|
while (--argc > 0) {
|
|
char *p = *++argv;
|
|
if (!strcmp(p, "-v")) {
|
|
really_verbose = TRUE;
|
|
} else if (!strcmp(p, "-g")) {
|
|
grade = TRUE;
|
|
} else if (*p == '-') {
|
|
fprintf(stderr, "%s: unrecognised option `%s'\n", argv[0], p);
|
|
return 1;
|
|
} else {
|
|
id = p;
|
|
}
|
|
}
|
|
|
|
if (!id) {
|
|
fprintf(stderr, "usage: %s [-g | -v] <game_id>\n", argv[0]);
|
|
return 1;
|
|
}
|
|
|
|
desc = strchr(id, ':');
|
|
if (!desc) {
|
|
fprintf(stderr, "%s: game id expects a colon in it\n", argv[0]);
|
|
return 1;
|
|
}
|
|
*desc++ = '\0';
|
|
|
|
p = default_params();
|
|
decode_params(p, id);
|
|
err = validate_desc(p, desc);
|
|
if (err) {
|
|
fprintf(stderr, "%s: %s\n", argv[0], err);
|
|
return 1;
|
|
}
|
|
s = new_game(NULL, p, desc);
|
|
s2 = new_game(NULL, p, desc);
|
|
|
|
sc = new_scratch(p->w, p->h);
|
|
|
|
/*
|
|
* When solving an Easy puzzle, we don't want to bother the
|
|
* user with Hard-level deductions. For this reason, we grade
|
|
* the puzzle internally before doing anything else.
|
|
*/
|
|
ret = -1; /* placate optimiser */
|
|
for (diff = 0; diff < DIFFCOUNT; diff++) {
|
|
ret = tents_solve(p->w, p->h, s->grid, s->numbers->numbers,
|
|
s2->grid, sc, diff);
|
|
if (ret < 2)
|
|
break;
|
|
}
|
|
|
|
if (diff == DIFFCOUNT) {
|
|
if (grade)
|
|
printf("Difficulty rating: too hard to solve internally\n");
|
|
else
|
|
printf("Unable to find a unique solution\n");
|
|
} else {
|
|
if (grade) {
|
|
if (ret == 0)
|
|
printf("Difficulty rating: impossible (no solution exists)\n");
|
|
else if (ret == 1)
|
|
printf("Difficulty rating: %s\n", tents_diffnames[diff]);
|
|
} else {
|
|
verbose = really_verbose;
|
|
ret = tents_solve(p->w, p->h, s->grid, s->numbers->numbers,
|
|
s2->grid, sc, diff);
|
|
if (ret == 0)
|
|
printf("Puzzle is inconsistent\n");
|
|
else
|
|
fputs(game_text_format(s2), stdout);
|
|
}
|
|
}
|
|
|
|
return 0;
|
|
}
|
|
|
|
#endif
|