mirror of
git://git.tartarus.org/simon/puzzles.git
synced 2025-04-20 23:51:29 -07:00
Files
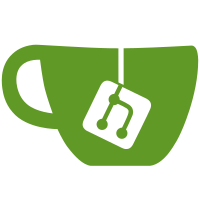
C89 provided only double-precision mathematical functions (sin() etc), and so despite using single-precision elsewhere, those are what Puzzles has traditionally used. C99 introduced single-precision equivalents (sinf() etc), and I hope it's been long enough that we can safely use them. Maybe they'll even be faster. Rather than directly use the single-precision functions, though, we use the magic macros from <tgmath.h> that automatically choose the precision of mathematical functions based on their arguments. This has the advantage that we only need to change which header we include, and thus that we can switch back again if some platform has trouble with the new header.
881 lines
27 KiB
C
881 lines
27 KiB
C
/*
|
|
* Code to generate patches of the aperiodic 'hat' tiling discovered
|
|
* in 2023.
|
|
*
|
|
* auxiliary/doc/hats.html contains an explanation of the basic ideas
|
|
* of this algorithm, which can't really be put in a source file
|
|
* because it just has too many complicated diagrams. So read that
|
|
* first, because the comments in here will refer to it.
|
|
*
|
|
* Discoverers' website: https://cs.uwaterloo.ca/~csk/hat/
|
|
* Preprint of paper: https://arxiv.org/abs/2303.10798
|
|
*/
|
|
|
|
#include <assert.h>
|
|
#include <tgmath.h>
|
|
#include <stdbool.h>
|
|
#include <stdio.h>
|
|
#include <stdlib.h>
|
|
#include <string.h>
|
|
|
|
#include "puzzles.h"
|
|
#include "hat.h"
|
|
#include "hat-internal.h"
|
|
|
|
void hat_kiteenum_first(KiteEnum *s, int w, int h)
|
|
{
|
|
Kite start = { {0,0}, {0, 3}, {3, 0}, {2, 2} };
|
|
size_t i;
|
|
|
|
for (i = 0; i < KE_NKEEP; i++)
|
|
s->recent[i] = start; /* initialise to *something* */
|
|
s->curr_index = 0;
|
|
s->curr = &s->recent[s->curr_index];
|
|
s->state = 1;
|
|
s->w = w;
|
|
s->h = h;
|
|
s->x = 0;
|
|
s->y = 0;
|
|
}
|
|
|
|
bool hat_kiteenum_next(KiteEnum *s)
|
|
{
|
|
unsigned lastbut1 = s->last_index;
|
|
s->last_index = s->curr_index;
|
|
s->curr_index = (s->curr_index + 1) % KE_NKEEP;
|
|
s->curr = &s->recent[s->curr_index];
|
|
|
|
switch (s->state) {
|
|
/* States 1,2,3 walk rightwards along the upper side of a
|
|
* horizontal grid line with a pointy kite end at the start
|
|
* point */
|
|
case 1:
|
|
s->last_step = KS_F_RIGHT;
|
|
s->state = 2;
|
|
break;
|
|
|
|
case 2:
|
|
if (s->x+1 >= s->w) {
|
|
s->last_step = KS_F_RIGHT;
|
|
s->state = 4;
|
|
break;
|
|
}
|
|
s->last_step = KS_RIGHT;
|
|
s->state = 3;
|
|
s->x++;
|
|
break;
|
|
|
|
case 3:
|
|
s->last_step = KS_RIGHT;
|
|
s->state = 1;
|
|
break;
|
|
|
|
/* State 4 is special: we've just moved up into a row below a
|
|
* grid line, but we can't produce the rightmost tile of that
|
|
* row because it's not adjacent any tile so far emitted. So
|
|
* instead, emit the second-rightmost tile, and next time,
|
|
* we'll emit the rightmost. */
|
|
case 4:
|
|
s->last_step = KS_LEFT;
|
|
s->state = 5;
|
|
break;
|
|
|
|
/* And now we have to emit the third-rightmost tile relative
|
|
* to the last but one tile we emitted (the one from state 2,
|
|
* not state 4). */
|
|
case 5:
|
|
s->last_step = KS_RIGHT;
|
|
s->last_index = lastbut1;
|
|
s->state = 6;
|
|
break;
|
|
|
|
/* Now states 6-8 handle the general case of walking leftwards
|
|
* along the lower side of a line, starting from a
|
|
* right-angled kite end. */
|
|
case 6:
|
|
if (s->x <= 0) {
|
|
if (s->y+1 >= s->h) {
|
|
s->state = 0;
|
|
return false;
|
|
}
|
|
s->last_step = KS_RIGHT;
|
|
s->state = 9;
|
|
s->y++;
|
|
break;
|
|
}
|
|
s->last_step = KS_F_RIGHT;
|
|
s->state = 7;
|
|
s->x--;
|
|
break;
|
|
|
|
case 7:
|
|
s->last_step = KS_RIGHT;
|
|
s->state = 8;
|
|
break;
|
|
|
|
case 8:
|
|
s->last_step = KS_RIGHT;
|
|
s->state = 6;
|
|
break;
|
|
|
|
/* States 9,10,11 walk rightwards along the upper side of a
|
|
* horizontal grid line with a right-angled kite end at the
|
|
* start point. This time there's no awkward transition from
|
|
* the previous row. */
|
|
case 9:
|
|
s->last_step = KS_RIGHT;
|
|
s->state = 10;
|
|
break;
|
|
|
|
case 10:
|
|
s->last_step = KS_RIGHT;
|
|
s->state = 11;
|
|
break;
|
|
|
|
case 11:
|
|
if (s->x+1 >= s->w) {
|
|
/* Another awkward transition to the next row, where we
|
|
* have to generate it based on the previous state-9 tile.
|
|
* But this time at least we generate the rightmost tile
|
|
* of the new row, so the next states will be simple. */
|
|
s->last_step = KS_F_RIGHT;
|
|
s->last_index = lastbut1;
|
|
s->state = 12;
|
|
break;
|
|
}
|
|
s->last_step = KS_F_RIGHT;
|
|
s->state = 9;
|
|
s->x++;
|
|
break;
|
|
|
|
/* States 12,13,14 walk leftwards along the upper edge of a
|
|
* horizontal grid line with a pointy kite end at the start
|
|
* point */
|
|
case 12:
|
|
s->last_step = KS_F_RIGHT;
|
|
s->state = 13;
|
|
break;
|
|
|
|
case 13:
|
|
if (s->x <= 0) {
|
|
if (s->y+1 >= s->h) {
|
|
s->state = 0;
|
|
return false;
|
|
}
|
|
s->last_step = KS_LEFT;
|
|
s->state = 1;
|
|
s->y++;
|
|
break;
|
|
}
|
|
s->last_step = KS_RIGHT;
|
|
s->state = 14;
|
|
s->x--;
|
|
break;
|
|
|
|
case 14:
|
|
s->last_step = KS_RIGHT;
|
|
s->state = 12;
|
|
break;
|
|
|
|
default:
|
|
return false;
|
|
}
|
|
|
|
*s->curr = kite_step(s->recent[s->last_index], s->last_step);
|
|
return true;
|
|
}
|
|
|
|
/*
|
|
* The actual tables.
|
|
*/
|
|
#include "hat-tables.h"
|
|
|
|
/*
|
|
* One set of tables that we write by hand: the permitted ways to
|
|
* extend the coordinate system outwards from a given metatile.
|
|
*
|
|
* One obvious approach would be to make a table of all the places
|
|
* each metatile can appear in the expansion of another (e.g. H can be
|
|
* subtile 0, 1 or 2 of another H, subtile 0 of a T, or 0 or 1 of a P
|
|
* or an F), and when we need to decide what our current topmost tile
|
|
* turns out to be a subtile of, choose equiprobably at random from
|
|
* those options.
|
|
*
|
|
* That's what I did originally, but a better approach is to skew the
|
|
* probabilities. We'd like to generate our patch of actual tiling
|
|
* uniformly at random, in the sense that if you selected uniformly
|
|
* from a very large region of the plane, the distribution of possible
|
|
* finite patches of tiling would converge to some limit as that
|
|
* region tended to infinity, and we'd be picking from that limiting
|
|
* distribution on finite patches.
|
|
*
|
|
* For this we have to refer back to the original paper, which
|
|
* indicates the subset of each metatile's expansion that can be
|
|
* considered to 'belong' to that metatile, such that every subtile
|
|
* belongs to exactly one parent metatile, and the overlaps are
|
|
* eliminated. Reading out the diagrams from their Figure 2.8:
|
|
*
|
|
* - H: we discard three of the outer F subtiles, in the symmetric
|
|
* positions index by our coordinates as 7, 10, 11. So we keep the
|
|
* remaining subtiles {0,1,2,3,4,5,6,8,9,12}, which consist of
|
|
* three H, one T, three P and three F.
|
|
*
|
|
* - T: only the central H expanded from a T is considered to belong
|
|
* to it, so we just keep {0}, a single H.
|
|
*
|
|
* - P: we discard everything intersected by a long edge of the
|
|
* parallelogram, leaving the central three tiles and the endmost
|
|
* pair of F. That is, we keep {0,1,4,5,10}, consisting of two H,
|
|
* one P and two F.
|
|
*
|
|
* - F: looks like P at one end, and we retain the corresponding set
|
|
* of tiles there, but at the other end we keep the two F on either
|
|
* side of the endmost one. So we keep {0,1,3,6,8,10}, consisting of
|
|
* two H, one P and _three_ F.
|
|
*
|
|
* Adding up the tile numbers gives us this matrix system:
|
|
*
|
|
* (H_1) (3 1 2 2)(H_0)
|
|
* (T_1) = (1 0 0 0)(T_0)
|
|
* (P_1) (3 0 1 1)(P_0)
|
|
* (F_1) (3 0 2 3)(F_0)
|
|
*
|
|
* which says that if you have a patch of metatiling consisting of H_0
|
|
* H tiles, T_0 T tiles etc, then this matrix shows the number H_1 of
|
|
* smaller H tiles, etc, expanded from it.
|
|
*
|
|
* If you expand _many_ times, that's equivalent to raising the matrix
|
|
* to a power:
|
|
*
|
|
* n
|
|
* (H_n) (3 1 2 2) (H_0)
|
|
* (T_n) = (1 0 0 0) (T_0)
|
|
* (P_n) (3 0 1 1) (P_0)
|
|
* (F_n) (3 0 2 3) (F_0)
|
|
*
|
|
* The limiting distribution of metatiles is obtained by looking at
|
|
* the four-way ratio between H_n, T_n, P_n and F_n as n tends to
|
|
* infinity. To calculate this, we find the eigenvalues and
|
|
* eigenvectors of the matrix, and extract the eigenvector
|
|
* corresponding to the eigenvalue of largest magnitude. (Things get
|
|
* more complicated in cases where there isn't a _unique_ eigenvalue
|
|
* of largest magnitude, but here, there is.)
|
|
*
|
|
* That eigenvector is
|
|
*
|
|
* [ 1 ] [ 1 ]
|
|
* [ (7 - 3 sqrt(5)) / 2 ] ~= [ 0.14589803375031545538 ]
|
|
* [ 3 sqrt(5) - 6 ] [ 0.70820393249936908922 ]
|
|
* [ (9 - 3 sqrt(5)) / 2 ] [ 1.14589803375031545538 ]
|
|
*
|
|
* So those are the limiting relative proportions of metatiles.
|
|
*
|
|
* So if we have a particular metatile, how likely is it for its
|
|
* parent to be one of those? We have to adjust by the number of
|
|
* metatiles of each type that each tile has as its children. For
|
|
* example, the P and F tiles have one P child each, but the H has
|
|
* three P children. So if we have a P, the proportion of H in its
|
|
* potential ancestry is three times what's shown here. (And T can't
|
|
* occur at all as a parent.)
|
|
*
|
|
* In other words, we should choose _each coordinate_ with probability
|
|
* corresponding to one of those numbers (scaled down so they all sum
|
|
* to 1). Continuing to use P as an example, it will be:
|
|
*
|
|
* - child 4 of H with relative probability 1
|
|
* - child 5 of H with relative probability 1
|
|
* - child 6 of H with relative probability 1
|
|
* - child 4 of P with relative probability 0.70820393249936908922
|
|
* - child 3 of F with relative probability 1.14589803375031545538
|
|
*
|
|
* and then we obtain the true probabilities by scaling those values
|
|
* down so that they sum to 1.
|
|
*
|
|
* The tables below give a reasonable approximation in 32-bit
|
|
* integers to these proportions.
|
|
*/
|
|
|
|
typedef struct MetatilePossibleParent {
|
|
TileType type;
|
|
unsigned index;
|
|
unsigned long probability;
|
|
} MetatilePossibleParent;
|
|
|
|
/* The above probabilities scaled up by 10000000 */
|
|
#define PROB_H 10000000
|
|
#define PROB_T 1458980
|
|
#define PROB_P 7082039
|
|
#define PROB_F 11458980
|
|
|
|
static const MetatilePossibleParent parents_H[] = {
|
|
{ TT_H, 0, PROB_H },
|
|
{ TT_H, 1, PROB_H },
|
|
{ TT_H, 2, PROB_H },
|
|
{ TT_T, 0, PROB_T },
|
|
{ TT_P, 0, PROB_P },
|
|
{ TT_P, 1, PROB_P },
|
|
{ TT_F, 0, PROB_F },
|
|
{ TT_F, 1, PROB_F },
|
|
};
|
|
static const MetatilePossibleParent parents_T[] = {
|
|
{ TT_H, 3, PROB_H },
|
|
};
|
|
static const MetatilePossibleParent parents_P[] = {
|
|
{ TT_H, 4, PROB_H },
|
|
{ TT_H, 5, PROB_H },
|
|
{ TT_H, 6, PROB_H },
|
|
{ TT_P, 4, PROB_P },
|
|
{ TT_F, 3, PROB_F },
|
|
};
|
|
static const MetatilePossibleParent parents_F[] = {
|
|
{ TT_H, 8, PROB_H },
|
|
{ TT_H, 9, PROB_H },
|
|
{ TT_H, 12, PROB_H },
|
|
{ TT_P, 5, PROB_P },
|
|
{ TT_P, 10, PROB_P },
|
|
{ TT_F, 6, PROB_F },
|
|
{ TT_F, 8, PROB_F },
|
|
{ TT_F, 10, PROB_F },
|
|
};
|
|
|
|
static const MetatilePossibleParent *const possible_parents[] = {
|
|
parents_H, parents_T, parents_P, parents_F,
|
|
};
|
|
static const size_t n_possible_parents[] = {
|
|
lenof(parents_H), lenof(parents_T), lenof(parents_P), lenof(parents_F),
|
|
};
|
|
|
|
/*
|
|
* Similarly, we also want to choose our absolute starting hat with
|
|
* close to uniform probability, which again we do by looking at the
|
|
* limiting ratio of the metatile types, and this time, scaling by the
|
|
* number of hats in each metatile.
|
|
*
|
|
* We cheatingly use the same MetatilePossibleParent struct, because
|
|
* it's got all the right fields, even if it has an inappropriate
|
|
* name.
|
|
*/
|
|
static const MetatilePossibleParent starting_hats[] = {
|
|
{ TT_H, 0, PROB_H },
|
|
{ TT_H, 1, PROB_H },
|
|
{ TT_H, 2, PROB_H },
|
|
{ TT_H, 3, PROB_H },
|
|
{ TT_T, 0, PROB_P },
|
|
{ TT_P, 0, PROB_P },
|
|
{ TT_P, 1, PROB_P },
|
|
{ TT_F, 0, PROB_F },
|
|
{ TT_F, 1, PROB_F },
|
|
};
|
|
|
|
#undef PROB_H
|
|
#undef PROB_T
|
|
#undef PROB_P
|
|
#undef PROB_F
|
|
|
|
HatCoords *hat_coords_new(void)
|
|
{
|
|
HatCoords *hc = snew(HatCoords);
|
|
hc->nc = hc->csize = 0;
|
|
hc->c = NULL;
|
|
return hc;
|
|
}
|
|
|
|
void hat_coords_free(HatCoords *hc)
|
|
{
|
|
if (hc) {
|
|
sfree(hc->c);
|
|
sfree(hc);
|
|
}
|
|
}
|
|
|
|
void hat_coords_make_space(HatCoords *hc, size_t size)
|
|
{
|
|
if (hc->csize < size) {
|
|
hc->csize = hc->csize * 5 / 4 + 16;
|
|
if (hc->csize < size)
|
|
hc->csize = size;
|
|
hc->c = sresize(hc->c, hc->csize, HatCoord);
|
|
}
|
|
}
|
|
|
|
HatCoords *hat_coords_copy(HatCoords *hc_in)
|
|
{
|
|
HatCoords *hc_out = hat_coords_new();
|
|
hat_coords_make_space(hc_out, hc_in->nc);
|
|
memcpy(hc_out->c, hc_in->c, hc_in->nc * sizeof(*hc_out->c));
|
|
hc_out->nc = hc_in->nc;
|
|
return hc_out;
|
|
}
|
|
|
|
static const MetatilePossibleParent *choose_mpp(
|
|
random_state *rs, const MetatilePossibleParent *parents, size_t nparents)
|
|
{
|
|
/*
|
|
* If we needed to do this _efficiently_, we'd rewrite all those
|
|
* tables above as cumulative frequency tables and use binary
|
|
* search. But this happens about log n times in a grid of area n,
|
|
* so it hardly matters, and it's easier to keep the tables
|
|
* legible.
|
|
*/
|
|
unsigned long limit = 0, value;
|
|
size_t i;
|
|
|
|
for (i = 0; i < nparents; i++)
|
|
limit += parents[i].probability;
|
|
|
|
value = random_upto(rs, limit);
|
|
|
|
for (i = 0; i+1 < nparents; i++) {
|
|
if (value < parents[i].probability)
|
|
return &parents[i];
|
|
value -= parents[i].probability;
|
|
}
|
|
|
|
assert(i == nparents - 1);
|
|
assert(value < parents[i].probability);
|
|
return &parents[i];
|
|
}
|
|
void hatctx_init_random(HatContext *ctx, random_state *rs)
|
|
{
|
|
const MetatilePossibleParent *starting_hat = choose_mpp(
|
|
rs, starting_hats, lenof(starting_hats));
|
|
|
|
ctx->rs = rs;
|
|
ctx->prototype = hat_coords_new();
|
|
hat_coords_make_space(ctx->prototype, 3);
|
|
ctx->prototype->c[2].type = starting_hat->type;
|
|
ctx->prototype->c[2].index = -1;
|
|
ctx->prototype->c[1].type = TT_HAT;
|
|
ctx->prototype->c[1].index = starting_hat->index;
|
|
ctx->prototype->c[0].type = TT_KITE;
|
|
ctx->prototype->c[0].index = random_upto(rs, HAT_KITES);
|
|
ctx->prototype->nc = 3;
|
|
}
|
|
|
|
static inline int metatile_char_to_enum(char metatile)
|
|
{
|
|
return (metatile == 'H' ? TT_H :
|
|
metatile == 'T' ? TT_T :
|
|
metatile == 'P' ? TT_P :
|
|
metatile == 'F' ? TT_F : -1);
|
|
}
|
|
|
|
static void init_coords_params(HatContext *ctx,
|
|
const struct HatPatchParams *hp)
|
|
{
|
|
size_t i;
|
|
|
|
ctx->rs = NULL;
|
|
ctx->prototype = hat_coords_new();
|
|
|
|
assert(hp->ncoords >= 3);
|
|
|
|
hat_coords_make_space(ctx->prototype, hp->ncoords + 1);
|
|
ctx->prototype->nc = hp->ncoords + 1;
|
|
|
|
for (i = 0; i < hp->ncoords; i++)
|
|
ctx->prototype->c[i].index = hp->coords[i];
|
|
|
|
ctx->prototype->c[hp->ncoords].type =
|
|
metatile_char_to_enum(hp->final_metatile);
|
|
ctx->prototype->c[hp->ncoords].index = -1;
|
|
|
|
ctx->prototype->c[0].type = TT_KITE;
|
|
ctx->prototype->c[1].type = TT_HAT;
|
|
|
|
for (i = hp->ncoords - 1; i > 1; i--) {
|
|
TileType metatile = ctx->prototype->c[i+1].type;
|
|
assert(hp->coords[i] < nchildren[metatile]);
|
|
ctx->prototype->c[i].type = children[metatile][hp->coords[i]];
|
|
}
|
|
|
|
assert(hp->coords[0] < 8);
|
|
}
|
|
|
|
HatCoords *hatctx_initial_coords(HatContext *ctx)
|
|
{
|
|
return hat_coords_copy(ctx->prototype);
|
|
}
|
|
|
|
/*
|
|
* Extend hc until it has at least n coordinates in, by copying from
|
|
* ctx->prototype if needed, and extending ctx->prototype if needed in
|
|
* order to do that.
|
|
*/
|
|
void hatctx_extend_coords(HatContext *ctx, HatCoords *hc, size_t n)
|
|
{
|
|
if (ctx->prototype->nc < n) {
|
|
hat_coords_make_space(ctx->prototype, n);
|
|
while (ctx->prototype->nc < n) {
|
|
TileType type = ctx->prototype->c[ctx->prototype->nc - 1].type;
|
|
assert(ctx->prototype->c[ctx->prototype->nc - 1].index == -1);
|
|
const MetatilePossibleParent *parent;
|
|
|
|
if (ctx->rs)
|
|
parent = choose_mpp(ctx->rs, possible_parents[type],
|
|
n_possible_parents[type]);
|
|
else
|
|
parent = possible_parents[type];
|
|
|
|
ctx->prototype->c[ctx->prototype->nc - 1].index = parent->index;
|
|
ctx->prototype->c[ctx->prototype->nc].index = -1;
|
|
ctx->prototype->c[ctx->prototype->nc].type = parent->type;
|
|
ctx->prototype->nc++;
|
|
}
|
|
}
|
|
|
|
hat_coords_make_space(hc, n);
|
|
while (hc->nc < n) {
|
|
assert(hc->c[hc->nc - 1].index == -1);
|
|
assert(hc->c[hc->nc - 1].type == ctx->prototype->c[hc->nc - 1].type);
|
|
hc->c[hc->nc - 1].index = ctx->prototype->c[hc->nc - 1].index;
|
|
hc->c[hc->nc].index = -1;
|
|
hc->c[hc->nc].type = ctx->prototype->c[hc->nc].type;
|
|
hc->nc++;
|
|
}
|
|
}
|
|
|
|
void hatctx_cleanup(HatContext *ctx)
|
|
{
|
|
hat_coords_free(ctx->prototype);
|
|
}
|
|
|
|
/*
|
|
* The actual system for finding the coordinates of an adjacent kite.
|
|
*/
|
|
|
|
/*
|
|
* Kitemap step: ensure we have enough coordinates to know two levels
|
|
* of meta-tiling, and use the kite map for the outer layer to move
|
|
* around the individual kites. If this fails, return NULL.
|
|
*/
|
|
static HatCoords *try_step_coords_kitemap(
|
|
HatContext *ctx, HatCoords *hc_in, KiteStep step)
|
|
{
|
|
hatctx_extend_coords(ctx, hc_in, 4);
|
|
hat_coords_debug(" try kitemap ", hc_in, "\n");
|
|
unsigned kite = hc_in->c[0].index;
|
|
unsigned hat = hc_in->c[1].index;
|
|
unsigned meta = hc_in->c[2].index;
|
|
TileType meta2type = hc_in->c[3].type;
|
|
const KitemapEntry *ke = &kitemap[meta2type][
|
|
kitemap_index(step, kite, hat, meta)];
|
|
if (ke->kite >= 0) {
|
|
/*
|
|
* Success! We've got coordinates for the next kite in this
|
|
* direction.
|
|
*/
|
|
HatCoords *hc_out = hat_coords_copy(hc_in);
|
|
|
|
hc_out->c[2].index = ke->meta;
|
|
hc_out->c[2].type = children[meta2type][ke->meta];
|
|
hc_out->c[1].index = ke->hat;
|
|
hc_out->c[1].type = TT_HAT;
|
|
hc_out->c[0].index = ke->kite;
|
|
hc_out->c[0].type = TT_KITE;
|
|
|
|
hat_coords_debug(" success! ", hc_out, "\n");
|
|
return hc_out;
|
|
}
|
|
|
|
return NULL;
|
|
}
|
|
|
|
/*
|
|
* Recursive metamap step. Try using the metamap to rewrite the
|
|
* coordinates at hc->c[depth] and hc->c[depth+1] (using the metamap
|
|
* for the tile type described in hc->c[depth+2]). If successful,
|
|
* recurse back down to see if this led to a successful step via the
|
|
* kitemap. If even that fails (so that we need to try a higher-order
|
|
* metamap rewrite), return NULL.
|
|
*/
|
|
static HatCoords *try_step_coords_metamap(
|
|
HatContext *ctx, HatCoords *hc_in, KiteStep step, size_t depth)
|
|
{
|
|
HatCoords *hc_tmp = NULL, *hc_out;
|
|
|
|
hatctx_extend_coords(ctx, hc_in, depth+3);
|
|
#ifdef HAT_COORDS_DEBUG
|
|
fprintf(stderr, " try meta %-4d", (int)depth);
|
|
hat_coords_debug("", hc_in, "\n");
|
|
#endif
|
|
unsigned meta_orig = hc_in->c[depth].index;
|
|
unsigned meta2_orig = hc_in->c[depth+1].index;
|
|
TileType meta3type = hc_in->c[depth+2].type;
|
|
|
|
unsigned meta = meta_orig, meta2 = meta2_orig;
|
|
|
|
while (true) {
|
|
const MetamapEntry *me;
|
|
HatCoords *hc_curr = hc_tmp ? hc_tmp : hc_in;
|
|
|
|
if (depth > 2)
|
|
hc_out = try_step_coords_metamap(ctx, hc_curr, step, depth - 1);
|
|
else
|
|
hc_out = try_step_coords_kitemap(ctx, hc_curr, step);
|
|
if (hc_out) {
|
|
hat_coords_free(hc_tmp);
|
|
return hc_out;
|
|
}
|
|
|
|
me = &metamap[meta3type][metamap_index(meta, meta2)];
|
|
assert(me->meta != -1);
|
|
if (me->meta == meta_orig && me->meta2 == meta2_orig) {
|
|
hat_coords_free(hc_tmp);
|
|
return NULL;
|
|
}
|
|
|
|
meta = me->meta;
|
|
meta2 = me->meta2;
|
|
|
|
/*
|
|
* We must do the rewrite in a copy of hc_in. It's not
|
|
* _necessarily_ obvious that that's the case (any successful
|
|
* rewrite leaves the coordinates still valid and still
|
|
* referring to the same kite, right?). But the problem is
|
|
* that we might do a rewrite at this level more than once,
|
|
* and in between, a metamap rewrite at the next level down
|
|
* might have modified _one_ of the two coordinates we're
|
|
* messing about with. So it's easiest to let the recursion
|
|
* just use a separate copy.
|
|
*/
|
|
if (!hc_tmp)
|
|
hc_tmp = hat_coords_copy(hc_in);
|
|
|
|
hc_tmp->c[depth+1].index = meta2;
|
|
hc_tmp->c[depth+1].type = children[meta3type][meta2];
|
|
hc_tmp->c[depth].index = meta;
|
|
hc_tmp->c[depth].type = children[hc_tmp->c[depth+1].type][meta];
|
|
|
|
hat_coords_debug(" rewritten -> ", hc_tmp, "\n");
|
|
}
|
|
}
|
|
|
|
/*
|
|
* The top-level algorithm for finding the next tile.
|
|
*/
|
|
HatCoords *hatctx_step(HatContext *ctx, HatCoords *hc_in, KiteStep step)
|
|
{
|
|
HatCoords *hc_out;
|
|
size_t depth;
|
|
|
|
#ifdef HAT_COORDS_DEBUG
|
|
static const char *const directions[] = {
|
|
" left\n", " right\n", " forward left\n", " forward right\n" };
|
|
hat_coords_debug("step start ", hc_in, directions[step]);
|
|
#endif
|
|
|
|
/*
|
|
* First, just try a kitemap step immediately. If that succeeds,
|
|
* we're done.
|
|
*/
|
|
if ((hc_out = try_step_coords_kitemap(ctx, hc_in, step)) != NULL)
|
|
return hc_out;
|
|
|
|
/*
|
|
* Otherwise, try metamap rewrites at successively higher layers
|
|
* until one works. Each one will recurse back down to the
|
|
* kitemap, as described above.
|
|
*/
|
|
for (depth = 2;; depth++) {
|
|
if ((hc_out = try_step_coords_metamap(
|
|
ctx, hc_in, step, depth)) != NULL)
|
|
return hc_out;
|
|
}
|
|
}
|
|
|
|
/*
|
|
* Generate a random set of parameters for a tiling of a given size.
|
|
* To do this, we iterate over the whole tiling via hat_kiteenum_first
|
|
* and hat_kiteenum_next, and for each kite, calculate its
|
|
* coordinates. But then we throw the coordinates away and don't do
|
|
* anything with them!
|
|
*
|
|
* But the side effect of _calculating_ all those coordinates is that
|
|
* we found out how far ctx->prototype needed to be extended, and did
|
|
* so, pulling random choices out of our random_state. So after this
|
|
* iteration, ctx->prototype contains everything we need to replicate
|
|
* the same piece of tiling next time.
|
|
*/
|
|
void hat_tiling_randomise(struct HatPatchParams *hp, int w, int h,
|
|
random_state *rs)
|
|
{
|
|
HatContext ctx[1];
|
|
HatCoords *coords[KE_NKEEP];
|
|
KiteEnum s[1];
|
|
size_t i;
|
|
|
|
hatctx_init_random(ctx, rs);
|
|
for (i = 0; i < lenof(coords); i++)
|
|
coords[i] = NULL;
|
|
|
|
hat_kiteenum_first(s, w, h);
|
|
coords[s->curr_index] = hatctx_initial_coords(ctx);
|
|
|
|
while (hat_kiteenum_next(s)) {
|
|
hat_coords_free(coords[s->curr_index]);
|
|
coords[s->curr_index] = hatctx_step(
|
|
ctx, coords[s->last_index], s->last_step);
|
|
}
|
|
|
|
hp->ncoords = ctx->prototype->nc - 1;
|
|
hp->coords = snewn(hp->ncoords, unsigned char);
|
|
for (i = 0; i < hp->ncoords; i++)
|
|
hp->coords[i] = ctx->prototype->c[i].index;
|
|
hp->final_metatile = tilechars[ctx->prototype->c[hp->ncoords].type];
|
|
|
|
hatctx_cleanup(ctx);
|
|
for (i = 0; i < lenof(coords); i++)
|
|
hat_coords_free(coords[i]);
|
|
}
|
|
|
|
const char *hat_tiling_params_invalid(const struct HatPatchParams *hp)
|
|
{
|
|
TileType metatile;
|
|
size_t i;
|
|
|
|
if (hp->ncoords < 3)
|
|
return "Grid parameters require at least three coordinates";
|
|
if (metatile_char_to_enum(hp->final_metatile) < 0)
|
|
return "Grid parameters contain an invalid final metatile";
|
|
if (hp->coords[0] >= 8)
|
|
return "Grid parameters contain an invalid kite index";
|
|
|
|
metatile = metatile_char_to_enum(hp->final_metatile);
|
|
for (i = hp->ncoords - 1; i > 1; i--) {
|
|
if (hp->coords[i] >= nchildren[metatile])
|
|
return "Grid parameters contain an invalid metatile index";
|
|
metatile = children[metatile][hp->coords[i]];
|
|
}
|
|
|
|
if (hp->coords[1] >= hats_in_metatile[metatile])
|
|
return "Grid parameters contain an invalid hat index";
|
|
|
|
return NULL;
|
|
}
|
|
|
|
void maybe_report_hat(int w, int h, Kite kite, HatCoords *hc,
|
|
internal_hat_callback_fn cb, void *cbctx)
|
|
{
|
|
Kite kite0;
|
|
Point vertices[14];
|
|
size_t i, j;
|
|
bool reversed = false;
|
|
int coords[28];
|
|
|
|
/* Only iterate from kite #0 of a hat */
|
|
if (hc->c[0].index != 0)
|
|
return;
|
|
kite0 = kite;
|
|
|
|
/*
|
|
* Identify reflected hats: they are always hat #3 of an H
|
|
* metatile. If we find one, reflect the starting kite so that the
|
|
* kite_step operations below will go in the other direction.
|
|
*/
|
|
if (hc->c[2].type == TT_H && hc->c[1].index == 3) {
|
|
reversed = true;
|
|
Point tmp = kite.left;
|
|
kite.left = kite.right;
|
|
kite.right = tmp;
|
|
}
|
|
|
|
vertices[0] = kite.centre;
|
|
vertices[1] = kite.right;
|
|
vertices[2] = kite.outer;
|
|
vertices[3] = kite.left;
|
|
kite = kite_left(kite); /* now on kite #1 */
|
|
kite = kite_forward_right(kite); /* now on kite #2 */
|
|
vertices[4] = kite.centre;
|
|
kite = kite_right(kite); /* now on kite #3 */
|
|
vertices[5] = kite.right;
|
|
vertices[6] = kite.outer;
|
|
kite = kite_forward_left(kite); /* now on kite #4 */
|
|
vertices[7] = kite.left;
|
|
vertices[8] = kite.centre;
|
|
kite = kite_right(kite); /* now on kite #5 */
|
|
kite = kite_right(kite); /* now on kite #6 */
|
|
kite = kite_right(kite); /* now on kite #7 */
|
|
vertices[9] = kite.right;
|
|
vertices[10] = kite.outer;
|
|
vertices[11] = kite.left;
|
|
kite = kite_left(kite); /* now on kite #6 again */
|
|
vertices[12] = kite.outer;
|
|
vertices[13] = kite.left;
|
|
|
|
if (reversed) {
|
|
/* For a reversed kite, also reverse the vertex order, so that
|
|
* we report every polygon in a consistent orientation */
|
|
for (i = 0, j = 13; i < j; i++, j--) {
|
|
Point tmp = vertices[i];
|
|
vertices[i] = vertices[j];
|
|
vertices[j] = tmp;
|
|
}
|
|
}
|
|
|
|
/*
|
|
* Convert from our internal coordinate system into the orthogonal
|
|
* one used in this module's external API. In the same loop, we
|
|
* might as well do the bounds check.
|
|
*/
|
|
for (i = 0; i < 14; i++) {
|
|
Point v = vertices[i];
|
|
int x = (v.x * 2 + v.y) / 3, y = v.y;
|
|
|
|
if (x < 0 || x > 4*w || y < 0 || y > 6*h)
|
|
return; /* a vertex of this kite is out of bounds */
|
|
|
|
coords[2*i] = x;
|
|
coords[2*i+1] = y;
|
|
}
|
|
|
|
cb(cbctx, kite0, hc, coords);
|
|
}
|
|
|
|
struct internal_ctx {
|
|
hat_tile_callback_fn external_cb;
|
|
void *external_cbctx;
|
|
};
|
|
static void report_hat(void *vctx, Kite kite0, HatCoords *hc, int *coords)
|
|
{
|
|
struct internal_ctx *ctx = (struct internal_ctx *)vctx;
|
|
ctx->external_cb(ctx->external_cbctx, 14, coords);
|
|
}
|
|
|
|
/*
|
|
* Generate a hat tiling from a previously generated set of parameters.
|
|
*/
|
|
void hat_tiling_generate(const struct HatPatchParams *hp, int w, int h,
|
|
hat_tile_callback_fn cb, void *cbctx)
|
|
{
|
|
HatContext ctx[1];
|
|
HatCoords *coords[KE_NKEEP];
|
|
KiteEnum s[1];
|
|
size_t i;
|
|
struct internal_ctx report_hat_ctx[1];
|
|
|
|
report_hat_ctx->external_cb = cb;
|
|
report_hat_ctx->external_cbctx = cbctx;
|
|
|
|
init_coords_params(ctx, hp);
|
|
for (i = 0; i < lenof(coords); i++)
|
|
coords[i] = NULL;
|
|
|
|
hat_kiteenum_first(s, w, h);
|
|
coords[s->curr_index] = hatctx_initial_coords(ctx);
|
|
maybe_report_hat(w, h, *s->curr, coords[s->curr_index],
|
|
report_hat, report_hat_ctx);
|
|
|
|
while (hat_kiteenum_next(s)) {
|
|
hat_coords_free(coords[s->curr_index]);
|
|
coords[s->curr_index] = hatctx_step(
|
|
ctx, coords[s->last_index], s->last_step);
|
|
maybe_report_hat(w, h, *s->curr, coords[s->curr_index],
|
|
report_hat, report_hat_ctx);
|
|
}
|
|
|
|
hatctx_cleanup(ctx);
|
|
for (i = 0; i < lenof(coords); i++)
|
|
hat_coords_free(coords[i]);
|
|
}
|